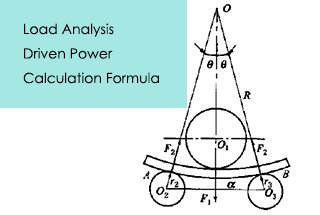
Have you ever wondered how massive steel plates are transformed into perfectly curved shapes? In this captivating blog post, we’ll dive into the fascinating world of 3-roll bending machines. Discover the ingenious working principles behind these mechanical marvels and gain insights from industry experts. Whether you’re an engineering enthusiast or simply curious about manufacturing processes, this article will unveil the secrets of precision metal bending. Get ready to be amazed by the power and precision of 3-roll bending machines!
As manufacturing continues to evolve rapidly, driven by national clean energy policies, there has been a significant surge in demand for coal-fired, hydropower, nuclear, and wind power generation facilities. This growth has consequently increased the need for large-scale plate rolling machines capable of processing pipeline components and column tower sections with precision and efficiency.
Concurrently, the expansion of offshore oil and gas exploration, petrochemical processing, and coal chemical industries has led to a rise in the production of heavy-duty high-pressure vessels. This trend has catalyzed the widespread adoption of specialized heavy-duty plate rolls designed to form thick, high-strength plates. These advanced rolling systems are crucial in fabricating critical components such as:
These plate rolling machines employ cutting-edge technologies to achieve the required dimensional accuracy, material properties, and surface finish. They often incorporate features such as variable crown control, work roll bending, and advanced automation systems to ensure consistent quality across a wide range of plate thicknesses and material grades, including high-strength low-alloy (HSLA) steels and specialized alloys used in extreme operating conditions.
The Roll Forming Machine, also known as the Plate Rolling Machine or Sheet Metal Roller, is a versatile metal forming equipment used to transform flat metal plates into cylindrical, conical, or curved shapes through a continuous bending process.
This machine operates on the principle of three-point bending, where the strategic positioning and synchronized rotation of the forming rolls induce controlled plastic deformation in the metal sheet. As the sheet passes through the rolls, it undergoes progressive bending, resulting in the desired profile of the workpiece.
Roll Forming Machines find extensive applications across various industries, including pressure vessel fabrication, naval architecture, oil and gas infrastructure, chemical processing plants, structural steel fabrication, and heavy machinery manufacturing.
In a typical 3-Roll configuration, two lower rolls function as drive rolls, capable of bi-directional rotation to facilitate both forward and reverse feeding. The upper roll, known as the pinch roll or bending roll, is vertically adjustable to control the bending radius and accommodate different material thicknesses. This arrangement allows for precise control over the forming process, enabling the production of complex geometries with high accuracy. (See figure)
Advanced models may incorporate features such as hydraulic or servo-electric actuation for enhanced precision, CNC controls for automated operation, and real-time monitoring systems to ensure consistent quality. Some machines also offer specialized attachments for tasks like cone rolling or edge pre-bending, further expanding their versatility in metal fabrication processes.
The process of rolling steel plate involves feeding the material between strategically positioned upper and lower rollers. The three-point contact created by these rollers induces controlled deformation, resulting in the plate’s transformation into a curved or circular profile.
This sheet metal forming technique can be conceptualized as a continuous three-point bending operation, executed by a precision three-roll bending machine. The process begins as one end of the metal plate is introduced between the upper and lower rollers.
The top roller exerts a calculated downward force on the metal plate, initiating plastic bending deformation through compressive stress. Simultaneously, the rotation of the lower rollers, driven by the frictional interface between the plate and roller surfaces, facilitates the plate’s bidirectional movement along its longitudinal axis.
As the plate traverses the roller deformation zone, plastic deformation occurs when the induced stress surpasses the material’s yield strength. This controlled deformation propagates along the entire length of the plate, resulting in a uniform plastic bending that conforms to the desired geometric profile.
The relative positioning of the upper and lower rolls can be precisely adjusted to achieve a wide range of bending radii. The minimum attainable radius is limited by the diameter of the upper roller, ensuring process flexibility while maintaining structural integrity.
A critical aspect of this process is the continuous application of downward pressure by the upper roller, which oscillates back and forth on the plate surface. This dynamic interaction ensures consistent deformation and contributes to the overall quality of the finished product.
The described method exemplifies the working principle of a symmetrical three-roll bending machine, a versatile tool in modern metal fabrication. Its ability to produce precise, repeatable results makes it invaluable in industries ranging from construction to aerospace.
Driven rollers II and III are powered by a synchronized motor-reducer system, rotating at identical speeds either in the same or opposite directions. This synchronization ensures uniform force distribution and consistent material flow during the bending process.
The plate’s forward movement is facilitated by the frictional forces generated between the rollers and the plate surface. This friction-based propulsion method allows for precise control of the plate’s progression through the bending apparatus.
The machine’s versatility in producing various curvatures is achieved through the precise adjustment of the upper roller’s position. This adjustability allows for fine-tuning of the bending radius to meet specific design requirements.
In cases where the desired curvature is not achieved in a single pass, the process employs an iterative approach. The upper roller’s position can be incrementally adjusted, and the rolling process repeated until the workpiece conforms to the specified shape. This adaptive methodology ensures high precision in the final product.
The symmetrical three-roller bending machine’s configuration, with rollers arranged in an isosceles triangle, introduces a characteristic straight-line segment at both ends of the workpiece during the rolling process. This unbent segment, approximately half the distance between the centers of the two lower rollers, represents a zone where effective rolling cannot occur and is considered the primary limitation of this machine type.
Despite this constraint, the symmetrical three-roller plate bending machine maintains widespread industrial adoption. Its popularity is attributed to its straightforward design, user-friendly operation, and cost-effectiveness, making it a preferred choice for many manufacturing applications.
To address the straight-line segment issue, various mitigation strategies can be employed, tailored to specific production requirements and workpiece characteristics. The following table outlines these methods, providing guidance for optimizing the bending process and minimizing end-effect imperfections.
Item | Solution |
Elbow pre-bending | This technique involves using a die to pre-bend the ends of the steel plate in a press machine, so as to achieve the desired curvature. |
Keep allowance | Add appropriate plate allowance at the ends of the plate. After rolling out a certain length at the two ends, the remainder (also known as the straight-line segment) can be cut off. |
Add base plate for pre-bending | This method is carried out on the rolling machine, as depicted in figure 3b. However, when adopting this method, it’s crucial to take into account the capacity of the rolling machine, that is, the combined bending force of the workpiece and the necessary gasket should not exceed that of the rolling machine. |
For advanced asymmetric three-roller bending machines, innovative shaft roller arrangements are designed to minimize or eliminate straight line segments on the rolled workpiece, enhancing the quality and precision of the final product.
The machine’s distinguishing feature is the vertical adjustability of both lower rollers. One lower roller can be precisely positioned to match the center distance of the upper roller, while the other is elevated to an optimal position based on the workpiece specifications and desired curvature. This dynamic configuration allows for greater control over the bending process and enables the production of more complex geometries.
The initial bending and rolling of the steel plate’s leading edge is facilitated by this adjustable setup. After completing approximately half a revolution, the alignment of the two lower rollers is strategically modified to continue the rolling process. This mid-process adjustment is crucial for eliminating the straight line segment typically formed at the trailing end of the workpiece, resulting in a more uniformly curved product.
Alternatively, for enhanced efficiency and consistency, the workpiece can be inverted after the initial pass. This technique positions the previously unbent end as the leading edge for a second rolling operation. This method not only eliminates the residual straight segment but also ensures a more symmetrical curvature throughout the entire length of the workpiece.
These advanced techniques, combined with precise CNC control and real-time feedback systems, allow for the production of high-quality curved components with minimal distortion and exceptional circular accuracy, meeting the stringent requirements of industries such as aerospace, energy, and large-scale industrial manufacturing.
Here is a brief overview of the process for rolling a short cylinder with a diameter of 400mm or greater, to help you understand the operation of a three-roller bending machine.
The material must be verified in accordance with the drawing and process requirements, and should have no obvious defects on its surface.
The material quality and specifications should conform to relevant national and industry standards.
When expanding, the cylinder’s diameter must match the actual diameter of the end enclosure, and the cylinder material’s diameter should be calculated based on the cylinder’s intermediate diameter.
The expanding direction should align with the steel rolling direction and be limited to a 45° position. The layout should be efficient, utilizing the edge material effectively and increasing the utilization of steel.
If the cylinder is constructed from multiple sections, the welding must be properly executed according to the technical requirements of equipment assembly and welding.
The spacing between butt welds of the end enclosure and longitudinal weld seams of the cylindrical shell section should be more than 3 times the cylinder’s thickness and not less than 100mm.
If the cylinder is connected to a pipe, support, reinforcing ring, base plate, etc., the interposition of the longitudinal and circumferential welds on the cylinder should prevent holes in the weld seam or being too close to it, and the reinforcing ring or base plate should cover the weld seams.
The line drawing should be precise, using a geometric mapping method to draw a vertical line, bisector, and midpoint, instead of a square master.
Allow for necessary margins, first draw the edge cutting line on the metal plate, then the actual material line, and verify the line.
Tolerance Requirements for Drawing Lines for Blanking:
The tolerance requirement for the line drawing of the cylinder height H is H ± 1mm.
The difference between two diagonal lines (△ L = L1 – L2) should be less than or equal to 2mm, and the length tolerance of the cylinder section is L ± 3mm.
The perimeter formula is L = π (Di + S), where Di is the cylinder diameter (mm) and S is the cylinder thickness (mm).
After marking, a material mark transplantation is made in the 100mm x 100mm box in the upper right corner of the steel plate.
Blanking and Edge Processing:
For carbon steel plates with a thickness less than 12mm, blanking is carried out using a shearing machine if possible (otherwise, a semi-automatic cutting machine is used).
After cutting, the slag should be cleaned and deburred.
For plates with a thickness greater than 6mm that require a groove, semi-automatic cutting machines or rolling chamfering machines are used. For plates with a thickness less than 6mm, the grinding method should be used.
The grooves generated by the flame cutting machine should have the slag cleaned, and the welding groove should not have flaws such as cracks or delaminations.
Before welding, the surface of the welded joint should be cleaned of any harmful impurities such as oxide, grease, and slag.
The clearance range (calculated from the groove or plate edge) should be ≥ 20mm.
When rolling a plate, the ends of the plate can be bent due to the lack of contact with the upper roller, resulting in residual straight edges.
During symmetrical bending, the residual straight edge is typically about half the center distance of the lower roller and depends on the plate thickness.
Asymmetrical bending results in residual straight edges that are approximately 1/6 to 1/10 of those in symmetrical bending.
These residual straight edges can be challenging to eliminate completely during correction and can lead to quality issues and equipment accidents, so they should be pre-bent.
If pre-bending is not possible, they can be corrected using a template after the final roll.
Before bending, the steel plate surface and roller surface must be cleaned, and any rust, leather, wool, edges, corners, or rigid particles must be removed.
For example, when rolling stainless steel, the upper and lower rollers must be protected by wrapping them in tape or a special paint layer, and the protective layer must not have any rigid particles.
When the plate is inserted into the rolling machine, to prevent misalignment, the workpiece should be rotated, and the workpiece’s main axis should be aligned parallel to the roller shaft to ensure that the round rolling is of good quality.
The circular rolling is the primary step in product forming and can be carried out in one-pass or multiple-pass processes.
The number of passes depends on the requirements of the process, such as the maximum permissible deformation rate in cold rolling, and the limitations of the equipment, such as the grip and power conditions.
A certain amount of over-rolling must be applied when the spring-back in cold rolling is significant.
The longitudinal stagger of the cylinder end should be less than 1.5mm. The plate rolling process is depicted in the accompanying figure.
The primary objective of roundness correction is to achieve uniform curvature throughout the entire circular profile, thereby enhancing product quality and dimensional accuracy. This process typically involves the following steps:
(1) Initial Setup and Feeding: Based on empirical data or precise calculations, adjust the rollers to the optimal correction curvature position. This initial setting is crucial as it determines the effectiveness of the subsequent rolling process. Consider factors such as material properties, thickness variations, and residual stresses from previous forming operations.
(2) Precision Round Rolling: Execute at least two complete rolling cycles under the determined correction curvature. Pay particular attention to the welding seam area, as this region often requires additional processing to ensure consistent curvature. Utilize advanced sensors and real-time monitoring systems to continuously assess and adjust the rolling parameters, ensuring uniform pressure distribution and material flow.
(3) Controlled Unloading: Gradually reduce the correction load in a controlled manner. Allow the workpiece to undergo several additional rolling cycles under progressively decreasing loads. This step is critical for stress relief and minimizing spring-back effects, resulting in improved dimensional stability of the final product.
Throughout the process, implement strict quality control measures, such as in-line laser measurement systems, to verify the achieved roundness against specified tolerances. For high-precision applications, consider integrating adaptive control algorithms that can dynamically adjust the rolling parameters based on real-time feedback, further optimizing the roundness correction process.