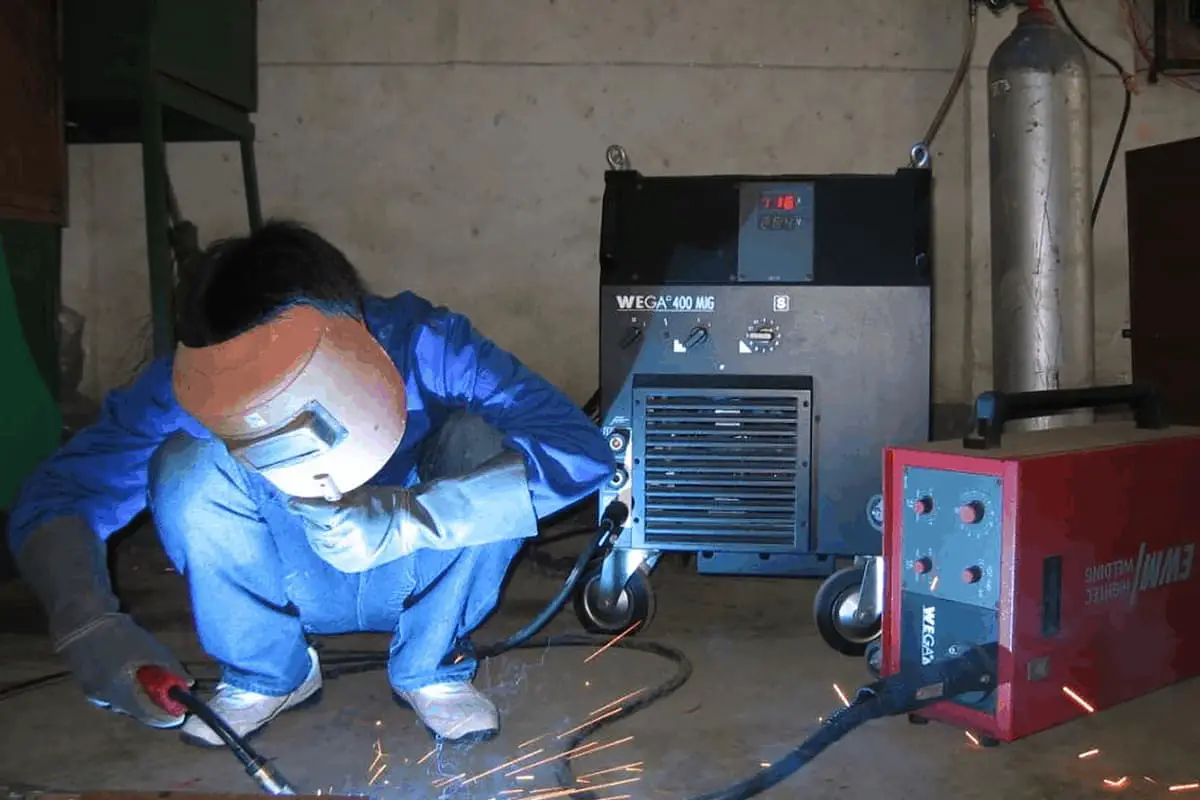
How do you get the perfect weld? Understanding the intricacies of adjusting welding machine current and voltage is crucial. This article explores the key factors influencing the ideal settings for CO2 gas shielded welding, including the relationship between welding current, voltage, and wire feeding speed. By mastering these elements, you can achieve a stable arc length and superior welding quality. Dive in to learn how to optimize your welding machine settings for the best results.
CO2 gas shielded welding, a type of Gas Metal Arc Welding (GMAW), utilizes an electric arc to generate heat, melting both the welding wire (electrode) and the base metal. The welding voltage, typically ranging from 15 to 35 volts, serves as the primary energy source, controlling the arc length and influencing the weld bead profile.
As the welding voltage increases, the arc length extends, resulting in a higher heat input and consequently a faster wire melting rate. This relationship is non-linear, with the melting rate increasing more rapidly at higher voltages due to increased resistive heating in the wire extension.
The welding current, typically ranging from 50 to 600 amperes depending on the application, is self-regulating in constant voltage systems. It’s determined by the dynamic equilibrium between the wire feed speed and the melting rate. The wire feeder continuously supplies wire at a preset speed, while the power source adjusts the current to maintain a stable arc length. This balance ensures consistent weld quality and penetration depth.
The selection of welding current is a critical parameter in achieving high-quality welds and is dependent on multiple factors including base metal thickness, welding position, travel speed, material composition, and joint configuration.
For Gas Metal Arc Welding (GMAW) using CO2 shielding gas, it is imperative to maintain a synergistic relationship between welding current, arc voltage, and wire feed speed. This balance is crucial for optimizing the melting rate of the electrode wire and ensuring consistent arc length stability throughout the welding process.
For a given welding wire, increasing the cable size results in a higher wire feeding speed.
Similarly, when the current remains constant, using a thinner welding wire will result in a faster wire feeding speed.
Welding voltage, also known as arc voltage, is a critical parameter that governs the energy input into the welding process. It plays a crucial role in determining the characteristics of the weld bead and overall weld quality.
The arc voltage directly influences the arc length and width, affecting the heat distribution across the weld pool. A higher arc voltage results in:
The effective arc voltage can be calculated by subtracting the voltage drop in the welding circuit from the output voltage of the power source. This relationship is expressed by the following equation:
Uarc = Uoutput – Uloss
Where:
Uarc = Arc voltage (V)
Uoutput = Power source output voltage (V)
Uloss = Voltage loss in the welding circuit (V)
In a properly installed welding setup that adheres to standard installation requirements, the primary source of voltage loss is typically attributed to cable extension. This loss is due to the electrical resistance of the extended cables.
When welding cables need to be extended to reach distant workpieces, it’s essential to compensate for the voltage drop. The output voltage of the welding machine should be adjusted according to the following guidelines:
Welding current Cable length | 100A | 200A | 300A | 400A | 500A |
10m | About 1V | About 1.5V | About 1V | About 1.5V | About 2V |
15m | About 1V | About 2.5V | About 2V | About 2.5V | About 3V |
20m | About 1.5V | About 3V | About 2.5V | About 3V | About 4V |
25m | About 2V | About 4V | About 3V | About 4V | About 5V |
It’s important to note that excessive cable length can lead to significant voltage drops, potentially compromising weld quality. Therefore, it’s recommended to use the shortest practical cable length and increase the cable cross-sectional area for long-distance applications to minimize voltage loss.
Selecting the appropriate welding voltage is crucial for achieving optimal weld quality and efficiency. The voltage is determined based on the welding current, which in turn depends on factors such as plate thickness, joint configuration, and welding position. Use the following formulas to calculate the recommended welding voltage range:
Example 1: Welding current of 200A (< 300A)
Welding voltage = (0.05 × 200 + 14 ± 2) V
= (10 + 14 ± 2) V
= 24 ± 2 V
Recommended voltage range: 22 – 26 V
Example 2: Welding current of 400A (≥ 300A)
Welding voltage = (0.05 × 400 + 14 ± 3) V
= (20 + 14 ± 3) V
= 34 ± 3 V
Recommended voltage range: 31 – 37 V
Note: These calculations provide a starting point for voltage settings. Fine-tuning may be necessary based on specific welding conditions, material properties, and desired weld characteristics. Always consult your welding procedure specification (WPS) and conduct test welds to ensure optimal results.
Welding voltage significantly impacts arc characteristics and weld bead geometry, directly affecting the overall welding quality. Optimal voltage selection is crucial for achieving desired weld properties and minimizing defects.
When the voltage is excessively high:
Conversely, when the voltage is too low:
Optimal voltage settings depend on factors such as welding process (e.g., GMAW, FCAW), wire feed speed, material thickness, and joint configuration. Modern welding power sources often feature synergic control, automatically adjusting voltage based on other parameters to maintain optimal arc characteristics.
Proper voltage selection, in conjunction with other welding parameters, is essential for achieving high-quality welds with minimal defects, optimal fusion, and desired mechanical properties.