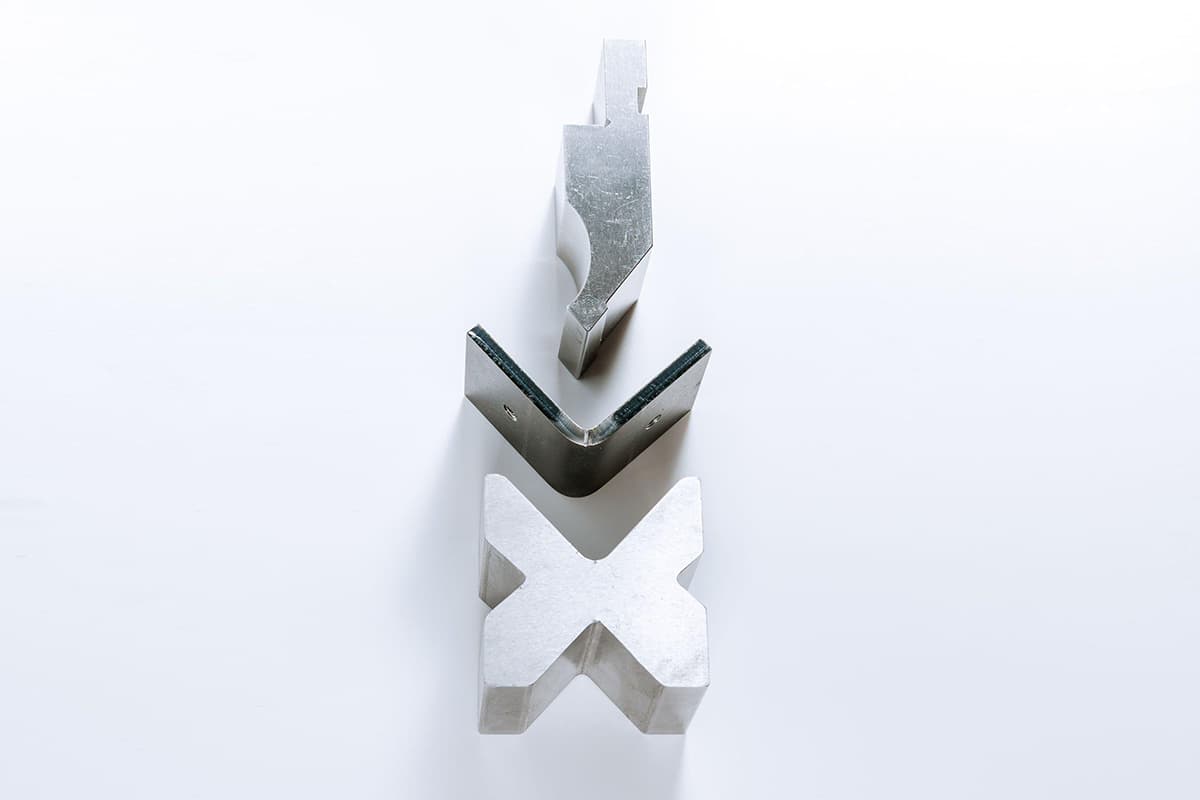
In this blog post, we’ll explore the fascinating world of air bending and the force charts that make it possible. Join us as we delve into the science behind this crucial process and discover how it shapes the products we use every day. Get ready to marvel at the ingenuity and precision that goes into every bend.
Our air bending force chart, based on the experience data of customers from various industries worldwide, provides an authoritative guide to help you easily determine the minimum bending pressure, minimum flange length, and bending radius corresponding to different V openings when bending mild steel and stainless steel.
With this chart, you can save time and increase efficiency in your bending process.
Additionally, our press brake tonnage calculator can assist you in calculating the required bending force for your specific sheet metal product.
We understand the importance of selecting the most appropriate V-opening, and our chart shows the optimum relationship between metal thickness and V-opening width.
Tonnage Requirements
Air bending is the preferred method of forming sheet material with a punch and die combination. Lower forming tonnage allows press brakes of lower capacity to manufacture the formed parts.
Figure below details the relationship between material thickness and die opening for a specified material tensile strength of 60,000 PSI. Note that as the tensile strength of most mild steels in North America exceed 60,000 PSI, higher tonnage values may be required.
Note:
The above chart illustrates the appropriate tonnage values to air bend mild steel with 60,000 PSI tensile properties. It must be noted that most North American steel mills are producing harder metals with typical mechanical properties of 44,000 PSI yield and up to 80,000 PSI tensile strengths. The tonnage values required to form these metals are substantially higher and must be taken into consideration in the selection of a press brake.
Die to Material Thickness Ratios
Standard industry practice for sizing the die opening is: eight times material thickness when less than 1/2”, ten times material thickness when 1/2” and greater.
For material in a heat treated condition, some material data sheets may specify larger die openings to prevent the formed shape from cracking.
For gauge sheet material and light plate, the punch radius is typically equal to the material thickness. For heavy plate, the punch radius is normally one and a half to three times the material thickness, depending on the properties of the plate being formed.
The air bending force chart records the standard lower die V width and the required bending force corresponding to the bending of different sheet metals and has become a general industry specification.
However, there was no such specification at first.
Each press brake manufacturer decided to use the V-width based on their own experience.
At that time, Amada collected and summarized the experience data of customers from various industries worldwide and finally made the following authoritative bending force chart for the bending process.
Through this bending force chart, you can easily determine the minimum bending pressure, minimum flange length, and bending radius corresponding to different V openings when bending mild steel and stainless steel.
To facilitate your reading and printing, you can download the PDF file of the air bending force chart.
You can also use our press brake tonnage calculator to calculate the required bending force for your sheet metal products.
Air bending is a method used in sheet metal fabrication to form metal into desired shapes and angles using a press brake. Unlike other bending techniques, air bending allows for greater flexibility and precision, as the metal is not pressed entirely to the bottom of the die. This process leaves a gap, or “air space,” between the metal and the die, providing better control over the bend angle and reducing the risk of over-bending or material deformation.
The primary tools used in air bending are the punch and die. The punch is the upper tool that presses down on the metal sheet, while the die is the lower tool with a V-shaped or U-shaped cavity that the metal bends into. For example, in the fabrication of brackets for automotive applications, a V-shaped die may be used to create precise angles that fit specific mounting requirements. The selection of the punch and die depends on factors such as the type of metal, its thickness, and the required bending angle.
The defining feature of air bending is the air gap between the metal and the bottom of the die. The punch stops before fully pressing the metal into the die, allowing for adjustments in the bend angle by varying the depth of the punch’s descent. This air gap helps maintain the integrity of the metal and prevents unwanted deformations. In scenarios where tight tolerances are required, such as in aerospace components, controlling the air gap is crucial to achieving the desired specifications.
Adding visual aids to illustrate these steps can enhance comprehension. For example, diagrams showing the alignment of the metal sheet, the descending punch, and the resulting bend can provide clarity on the process.
Air bending offers several advantages over other bending methods, such as bottom bending or coining.
You can get the following information from the air bending force chart above if the data of the metal thickness and bending inner raidus are known:
The V-opening refers to the distance across the lower die mouth, and the appropriate V-opening of the die should be selected according to the sheet metal thickness. The air bending chart above shows the optimum relationship between the metal thickness and V-opening width.
However, other factors will affect the selection of the V-width, including flange lengths, inner bending radius, press brake tonnage, and the capacity of the tooling.
Material Thickness (t)mm | 0.5-2.5 | 3.0-8.0 | 9.0-10.0 | ≥12.0 |
V-Width | 6xt | 8xt | 10xt | 12xt |
Air bending in sheet metal fabrication is a technique used to create bends in metal sheets without the metal making full contact with the die. In this process, a press brake equipped with a punch and a V-shaped or U-shaped die is used. The punch presses down on the sheet metal, pushing it into the die, but stops before reaching the bottom, leaving a gap between the metal and the die. This gap, or “air gap,” allows for flexibility in achieving various bend angles without the metal conforming entirely to the shape of the die.
The key components involved in air bending include the punch and die, which determine the shape and size of the bend, and the air gap, which provides the flexibility needed for different angles and radii. The process begins with setting up the punch and die according to the type, thickness, and required bending angle of the metal sheet. The sheet is then positioned on the die and aligned with the punch. As the punch descends, it bends the metal within the die, stopping short of the bottom to create the air gap.
Air bending offers several advantages, such as flexibility in producing a range of bend angles with the same die setup, faster production runs, reduced tooling costs, and better control over the bending process. However, it also has some disadvantages, including less accuracy compared to bottom bending and the potential for springback, where the metal partially returns to its original shape after bending. This springback can be managed by overbending or using adaptive forming technology.
Overall, air bending is a versatile and efficient method suitable for production runs where high precision is not critical, and for creating parts with varying bend angles without the need for frequent die changes.
To calculate the bending force required for air bending in metalworking, you need to consider several key factors, including material properties, geometry of the bend, and tooling used. The bending force ( F ) can be calculated using the following formula:
Where:
For example, if you are bending mild steel with a tensile strength of 45 kg/mm², a thickness of 2 mm, a bend length of 100 mm, and using a die opening of 6 mm, the calculation would be:
Additional considerations include the internal radius and minimum bend radius. The internal radius can be estimated using the formula:
For precise calculations, especially with different materials, consider:
Where ( TS_{\text{baseline}} ) is often 60,000 PSI for mild steel. The minimum bend radius ensures the bend does not cause cracks, calculated as:
Utilizing air bending force charts and calculators simplifies the process by providing pre-calculated values for common materials and die openings. These tools allow users to input specific parameters and obtain the required bending force directly, ensuring safe and precise metalworking operations.
Several factors affect the minimum bendable flange length in air bending, particularly when using an Air Bending Force Chart.
First, the material thickness is crucial; generally, the flange length should be at least four times the material thickness. For example, a 2mm thick sheet would require a minimum flange length of 8mm.
Second, the bend radius, which is determined by the V opening in the die, also influences the flange length. A larger bend radius typically necessitates a longer flange length to ensure proper bending.
Third, the die opening and tooling geometry impose minimum bend dimensions. The flange length must be sufficient to reach the top of the die after forming.
Additionally, while the K-factor itself doesn’t directly determine flange length, it is essential for calculating the bend allowance, which affects the overall flat pattern and, consequently, the flange length.
A common formula used to determine the minimum acceptable flange height is:
Furthermore, the minimum hole distance from the bend, though not directly related to flange length, is important to prevent deformation and indirectly influences flange design.
Finally, adhering to industry guidelines and tolerances, such as a +/- 1 degree tolerance on bend angles and consistent bend radii, helps maintain uniformity and ensures adequate flange lengths for successful bending.
By considering these factors, designers and manufacturers can accurately determine the minimum bendable flange length, leading to successful and consistent sheet metal fabrication.
The die opening (V) in air bending plays a crucial role in determining several key aspects of the bending process. Firstly, it directly influences the inside bend radius, with a larger V-die opening resulting in a larger bend radius. This is typically around 16-20% of the die opening width for materials like 60 KSI cold-rolled steel and stainless steel.
A common guideline, known as the “Rule of Eight,” suggests that the V-die opening should be eight times the material thickness, though this can vary between 6 to 12 times depending on specific conditions. The size of the die opening also affects the stress distribution within the material; a smaller die opening creates a tighter bend radius, increasing tensile stress on the outer layer and compressive stress on the inner layer, which can lead to material deformation or cracking if the stresses exceed the material’s strength.
Springback, the tendency of the material to partially revert to its original shape after bending, is also influenced by the V-die opening. A larger die opening usually results in more significant springback, necessitating over-bending to achieve the desired angle. Furthermore, the die opening impacts the tonnage or force required for bending, with thicker materials and smaller radii needing more force.
Lastly, the precision and productivity of the bending process are affected by the V-die opening. Air bending, which uses a V-die, is generally less precise than bottoming or coining but offers higher productivity and flexibility, as it requires fewer tool changes and can produce different profiles with adjustable tools.
Air bending can be used for a wide variety of materials, but its suitability depends on the specific properties of each material. It is effective for materials such as aluminum, stainless steel, carbon steel, copper, and some plastics. For instance, aluminum is highly malleable and formable, making it a good candidate for air bending. Stainless steel, while also suitable, exhibits a higher degree of springback, necessitating adjustments to the bending process. Carbon steel can be air-bent as well, though variations in thickness and grain direction need to be accounted for.
However, there are limitations to consider. Air bending generally requires less force than other bending methods like coining but can be less precise. The choice of tooling and die opening is crucial to achieving the desired bend angle and radius. Variations in material thickness can significantly impact the bend angle, and springback is a common issue that varies by material. Additionally, bending perpendicular to the grain direction can reduce the risk of cracks and deformation.
In conclusion, while air bending is versatile and can accommodate a range of materials, it is not universally applicable. The success of air bending hinges on the careful selection of materials, proper tooling, and a thorough understanding of the material’s properties. For applications requiring high precision or tight tolerances, other methods may be more appropriate.
When performing air bending operations on a press brake machine, several critical safety precautions must be taken to ensure the safety of the operators and the integrity of the equipment. First, operators should always wear appropriate Personal Protective Equipment (PPE), including safety goggles, gloves, and work boots, to protect against injuries from sharp metal edges and other hazards.
Before starting the machine, it is essential to inspect it thoroughly. Check electric connections, hydraulic fluid levels, and inspect for any damage such as cracks or breaks. Regular maintenance of the equipment, including hydraulic systems, is crucial to prevent accidents. Operators should read and understand the instruction manual to become familiar with the machine’s functioning and principles.
The work area should be kept clear of unnecessary personnel and equipment to reduce the risk of accidents. Proper placement of the sheet metal, parallel to the bending machine, is crucial for safe operation. Incorrect placement can lead to the sheet hitting the operator and causing injury.
Using guardrails to isolate the bending work area and implementing visual management for safety conditions are recommended. Modern safeguarding devices like light curtains or Active Optoelectronic Protective Devices (AOPD) can prevent operators from getting too close to the danger zone, stopping the machine if necessary.
Performing regular risk assessments and ensuring operators are trained, preferably by an experienced engineer, before using the machine for the first time is essential. Electrical safety measures, such as ensuring the equipment has a protective earth (PE) connection and avoiding contact with electrical equipment with wet hands, are critical.
Additional precautions include avoiding stacking materials too high, keeping the work surface clean, and handling wet metal sheets with care due to their slippery nature. Ensuring the machine is well-lubricated before use can also prevent damage to the rolls or material.
By following these safety precautions, operators can significantly reduce the risks associated with air bending operations and maintain a safe and efficient working environment.