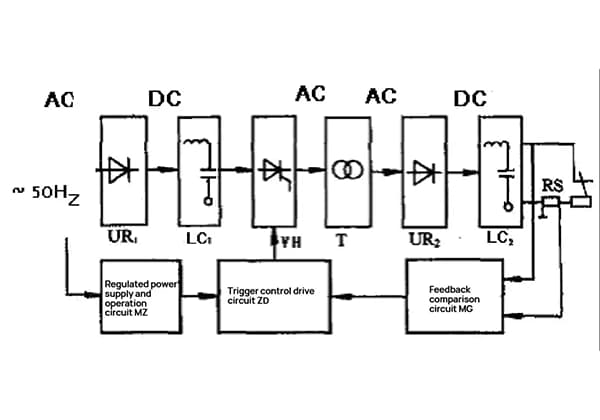
Have you ever wondered how modern welding achieves such precision and efficiency? Arc welding inverters might hold the key. These devices transform electrical energy to create powerful welding arcs, adapting dynamically to complex welding conditions. In this article, you’ll discover how these inverters work, their main components, and the principles behind their operation. By the end, you’ll understand how arc welding inverters enhance welding performance, making them indispensable in various industrial applications. Dive in to explore the technological marvels that make precision welding possible.
The definition of an arc welding inverter:
The transformation between direct current (DC) and alternating current (AC) is called inversion. The device that accomplishes this transformation is called an inverter. An inverter that provides electrical energy for welding arcs and has the required electrical performance for arc welding processes is called an arc welding inverter.
The special nature of arc welding inverter:
The power supply object for welding is a special arc load, especially for arc welding with short-circuit transition, which requires the inverter to withstand the intense dynamic load that is constantly changing. The working conditions are very complex.
The main components include the power supply system, electronic power system, electronic control system, feedback circuit, given circuit, and welding arc.
From the diagram, it can be seen that the main components and their functions of the arc welding inverter are as follows:
Inverter main circuit: Consisting of the power supply system, electronic power system, and welding arc, it is responsible for the transmission and conversion of electrical energy.
Electronic control system: Provides sufficient switch pulse signals to the electronic power system (inverter main circuit) according to the variation law required by the arc, driving the operation of the inverter main circuit.
Feedback and given system: It consists of detection circuit (M), given circuit (G), comparison and amplification circuit (N), etc. Together with the electronic control system, it realizes the closed-loop control of the arc welding inverter, and enables it to obtain the required external and dynamic characteristics.
The basic principle of arc welding inverters can be summarized in the block diagram shown in Figure 1.
In the power supply system, single-phase (or three-phase) 50Hz or 60Hz AC network voltage of 220V (or three-phase 380V) is rectified and filtered by the input rectifier (UR1) and filter (LC1), obtaining a smooth DC voltage of about 310V (or about 520V for three-phase rectification), which is required by the inverter main circuit.
The DC voltage is then converted into high-frequency AC voltage ranging from several kilohertz to two hundred kilohertz by the alternating switching action of the high-power switching electronic devices (such as thyristors, transistors, field effect transistors or IGBT) in the inverter main circuit Q of the electronic power system.
After that, the voltage is stepped down to tens of volts suitable for welding through the high (medium) frequency transformer (T), and then the external and dynamic characteristics required by the arc welding process are obtained through the control driving circuit and the feedback and given circuit (M, G, N, etc.) of the electronic control system, as well as the impedance of the welding circuit.
If direct current is required for welding, the high (medium) frequency AC is converted into DC output by the output rectifier U and the filter of the inductance L2 and capacitor C2.
The rectification process of arc welding inverters can be simply described as follows: AC input → rectification to DC → high/medium frequency AC conversion → voltage reduction → AC output → rectification to DC again.
There are three types of inverter structures that can be used in arc welding inverters:
In order to meet the requirements of arc welding process, the electrical output characteristics (performance) of arc welding inverters must have corresponding adaptability. The electrical output characteristics mainly include external characteristics, regulation performance, and dynamic characteristics.
Arc welding inverters use electronic control systems and current-voltage feedback to perform closed-loop control of the electronic power system (inverter), in order to obtain different external characteristic curve shapes.
Based on the basic principle block diagram of the arc welding inverter (Figure 1), the closed-loop control system of the arc welding inverter can be described using block diagrams and equations, as shown in Figure 2.
The balance relationship of the closed-loop control system is established as follows: The arc voltage (Uf) is negatively fed back in the diagram, and the output voltage is sampled (usually by a voltage divider) to obtain a feedback quantity (mUf) proportional to it. The arc current (If) is also negatively fed back, and the output current is sampled (usually by a shunt or Hall element) to obtain a feedback quantity (nIf) proportional to it. The feedback quantities mUf and nIf are then compared and amplified with the arc voltage set value (Ugu) and the arc current set value (Ugi) respectively, resulting in K1(Ugu-mUf) and K2(Ugi-n) outputs. Finally, the control voltage (Uk) is obtained through synthesis and amplification, and then input to the control drive circuit to drive the electronic power system (inverter) operation.
Obtaining the constant voltage, constant current, and soft dropping characteristics:
1 – Constant voltage characteristic
2 – Constant current characteristic
3 – Soft dropping characteristic
4 – Constant current with external dragging characteristic
From the principle of the external characteristic curve of the arc welding inverter, it can be inferred that for a given voltage value of the constant voltage characteristic, the size of the output arc voltage is determined. In other words, if the given voltage is high, the arc voltage is also high, and vice versa. For example, if Ugu1 < Ugu2, the external characteristic curve moves from curve 1 to curve 2, as shown in Figure 4a, and the stable operating point moves from A1 to A2.
For the constant current characteristic, the size of the voltage value for a given current determines the size of the output welding current. In other words, if Ugi is large, the output welding current is also large, and vice versa. For example, if Ugi1 < Ugi2, the external characteristic curve moves from curve 1 to curve 2, as shown in Figure 6-4b, and the stable operating point moves from AI to A2.
Generally, different types of arc welding inverters adopt different regulation systems to achieve control of the external characteristics and adjustment of process parameters to meet the different requirements of the welding process. We will introduce the working principles of different types of arc welding inverters one by one.
When arc welding inverters are used for arc welding processes with short circuit transitions involving molten droplets, strict requirements must be placed on their dynamic characteristics. The main parameter that affects the short circuit transition of MAG/CO2 welding is the rate of rise of short circuit current (disd/dt), which is directly related to the time constant T (T=L’/Rf, where L’ is the equivalent inductance of the welding circuit, and Rf is the arc resistance). Rf varies with the welding current and cannot be arbitrarily changed, while L’ can be changed by adding inductors to the welding circuit. In addition, the disd/dt can be changed by adjusting the time constant of the closed-loop system.
There are generally two ways to improve and control the dynamic characteristics of arc welding inverters:
Typically, arc welding inverters use three adjustment control modes to control external characteristics, adjustment characteristics (process parameter adjustment), and form output pulse waveforms:
Several commonly used basic forms of inverter main circuits are shown in Figure 6.
a) Single-end forward type
b) Half-bridge type
c) Full-bridge type
d) Parallel type.
Single-end forward inverter main circuit:
As shown in Figure 6a, power switching transistors (represented by electronic switch symbols) V1 and V2 are periodically turned on and off at the intermediate frequency, thereby inverting the input DC voltage into intermittent intermediate frequency voltage. The voltage is then stepped down by the intermediate frequency transformer T, rectified by the fast diode VD1, filtered by the inductor, and output as DC voltage to the arc. The two switching transistors simultaneously bear the input voltage, requiring a relatively low voltage resistance, making it suitable for medium and small power inverters.
Half-bridge inverter main circuit:
As shown in Figure 6b, the input DC voltage is divided equally by two sets of electrolytic capacitors. The two power switching transistors V1 and V2 are switched on and off alternately to form a rectangular waveform AC voltage.
After being stepped down by T, the full-wave rectification by VD1 and VD2 produces DC output. VD1 and VD2 must be fast diodes capable of withstanding double the output voltage amplitude. V1 and V2 only bear 1Ud/2, and have relatively low voltage resistance requirements.
Full-bridge inverter main circuit:
As shown in Figure 6c, two pairs of power switching transistors V1, V4 and V2, V3 on opposite bridge arms are periodically switched on and off at intermediate frequency. The rest of the operation is the same as the half-bridge. Power switching transistors also only bear a certain voltage, making it suitable for medium to high power welding requirements.
Parallel inverter main circuit:
As shown in Figure 6d, this type of main circuit is also known as a push-pull inverter main circuit. Power switching transistors V1 and V2 are periodically switched on and off at intermediate frequency.
After being stepped down by T, VD1 and VD2 perform full-wave rectification to output DC voltage. Switching transistors bear more than twice the voltage, requiring high voltage resistance. Generally, it is only used in thyristor-type inverters.
The electronic control system of an arc welding inverter actually includes both electronic control circuits and drive circuits. They are another important component for achieving the electrical performance of the arc welding inverter. Therefore, it is necessary to have a deep understanding of the functional requirements for these circuits and how to better fulfill these requirements.
The role of electronic control circuits is to provide a pair of rectangular pulse trains (excluding thyristor inverters) with steep leading and trailing edges, a phase difference of 180°, symmetry, and variable width or phase shift to the drive circuit of the arc welding inverter.
For some inverters, such as half-bridge and full-bridge inverters, the pulse trains must be isolated from each other. For a single-ended inverter, only one set of pulses is needed.
The design goal is achieved through the relationship between the presence or absence of paired pulse voltages, narrow and wide pulses, the amount of change in pulse width, or changing pulse frequency or phase, as well as the relationship between the basic pulse width, the minimum pulse width, and the speed at which the pulse width increases from the minimum to the rated width, and the relationship between the minimum and rated pulse frequency.
More specifically, the control circuit must have the following basic functions:
Other functions:
The pulse control signals provided by the control circuit must have sufficient power. However, due to the different types, models, and capacities of switching tubes, the power requirements for the drive pulse signals also differ.
Different types of inverter main circuits also have different isolation requirements for the drive pulse signals.
For example, in full-bridge and half-bridge inverter main circuits, the switching tubes located at high and low potentials require reliable isolation of the drive pulse signals.
The drive circuits for thyristor-based and transistor-based inverters have different characteristics and requirements.
Requirements for the drive circuit of thyristor-based inverters:
Requirements for the drive circuit of transistor-based inverters:
The role of the drive circuit for transistor-based inverters is to amplify the pulse output of the control circuit to a level sufficient to excite high-voltage switching tubes. The amplitude and waveform of the drive pulse provided are related to the operating characteristics of the transistor, such as saturation voltage drop, storage time, voltage and current rise and fall rate of the collector or emitter at the moment of opening and closing, which directly affect its loss and heat generation.
The drive circuit is one of the main factors determining the performance of PWM inverters.
Compared to traditional arc welding power sources which use a frequency of 50Hz or 60Hz to transmit energy and change electrical parameters, arc welding inverters increase the frequency to several thousand to two hundred thousand Hz for energy transmission and conversion.
This increase in frequency provides arc welding inverters with outstanding features in terms of structure and performance, including high efficiency and energy savings, lightweight and material-saving design, fast dynamic response, and excellent electrical and welding process performance.
Specifically, when compared to traditional arc welding power sources such as arc welding transformers, DC arc welding generators, silicon arc welding rectifiers, and thyristor arc welding rectifiers, arc welding inverters have the following significant characteristics and advantages:
Arc welding inverters can be classified in different ways:
Related reading: Types Of Arc Welding Inverters
Due to its excellent electrical performance, good control performance, ability to obtain various shapes of output characteristics, different types of arc voltage and current waveforms (DC, pulse, rectangular wave AC), and excellent dynamic characteristics, arc welding inverters can output welding currents of up to 1000A or more.
Therefore, it can almost replace all existing arc welding power sources and be used for various arc welding methods such as manual metal arc welding, TIG welding, MAG/C02/MIG/flux-cored wire welding, plasma arc welding and cutting, submerged arc automatic welding, robot welding, and others.
It can weld various metal materials and alloys, especially in applications with limited workspace, high altitude operations, or where there is a shortage of electrical supply and a need for mobile welding machines.