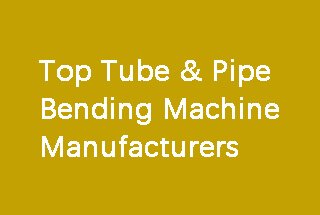
Have you ever marveled at the graceful curves of a stainless steel handrail or the sleek lines of a metal sculpture? Behind these beautiful designs lies the art and science of bending stainless steel tubing. In this article, we’ll explore the fascinating world of tube bending, guided by the insights of seasoned experts in the field. Discover the techniques, challenges, and innovations that shape this crucial aspect of modern manufacturing.
Stainless steel tubing is extensively utilized across diverse industries due to its exceptional corrosion resistance, high strength-to-weight ratio, and aesthetic appeal. The material properties of stainless steel are highly dependent on factors such as alloy composition, microstructure, and thermomechanical processing history. A comprehensive understanding of these properties is critical for optimizing bending processes and ensuring product integrity.
Grades: Common stainless steel grades for tubing applications include austenitic (304, 316), ferritic (409, 439), and duplex (2205) types. Each grade exhibits distinct mechanical and metallurgical characteristics that significantly impact formability:
Hardness: The hardness of stainless steel, typically measured in Rockwell B or C scales, directly influences the required bending force and the potential for strain-induced martensite formation. Annealed stainless steel (e.g., 304 with RB 70-80) offers improved formability compared to cold-worked variants (RB 80-95). However, work hardening during bending can significantly increase local hardness, necessitating process adjustments.
Ductility: Ductility, quantified by elongation percentage and reduction in area during tensile testing, is crucial for successful bending operations. Austenitic grades generally offer superior ductility (40-60% elongation) compared to ferritic types (20-30%). Higher ductility facilitates more severe bending radii and reduces the risk of cracking or orange-peeling effects. However, the strain rate sensitivity of some grades (particularly austenitic) must be considered in high-speed bending processes.
Yield Strength and Tensile Strength: The yield strength (YS) and ultimate tensile strength (UTS) of stainless steel tubing significantly affect springback behavior and required bending forces. Typical values for annealed 304 are:
Higher strength grades or cold-worked materials will require greater bending forces and exhibit more pronounced springback.
Anisotropy: Stainless steel tubing often exhibits anisotropic behavior due to the manufacturing process (e.g., drawing, welding). This can lead to variations in bending properties depending on the orientation relative to the tube axis. Consideration of the Lankford coefficient (r-value) can help predict and compensate for these effects during bending operations.
Strain Hardening: The strain hardening exponent (n-value) of stainless steel, particularly for austenitic grades, is relatively high. This characteristic allows for excellent stretch formability but can lead to rapid work hardening during bending. Progressive bending techniques or intermediate annealing may be necessary for severe forming operations.
Various bending techniques can be employed to shape stainless steel tubing, each with its own advantages and limitations. The selection of an appropriate method depends on factors such as desired bend radius, material properties, production volume, and end-use application. Here are some common methods:
Mandrel Bending: This precision technique utilizes a flexible or solid mandrel inserted into the tubing to maintain its internal geometry during bending. The mandrel prevents tube flattening, wrinkling, or collapse, ensuring consistent wall thickness and cross-sectional integrity. Mandrel bending is ideal for producing tight radius bends (down to 1D) and maintaining strict tolerances. It’s widely used in aerospace, automotive, and high-performance industrial applications where structural integrity and fluid flow characteristics are critical.
Rotary Draw Bending: This versatile method employs a rotating bend die, pressure die, and clamping block to create precise, repeatable bends. It offers excellent control over the bending process, minimizing material thinning and ovalization. Rotary draw bending is suitable for a wide range of bend radii and wall thicknesses, making it a popular choice for complex tubular components in industries such as HVAC, furniture, and medical equipment manufacturing.
Roll Bending: Also known as pyramid rolling, this technique uses three rollers (typically arranged in a triangular configuration) to apply pressure on the tubing, creating a smooth, continuous bend. The process is ideal for large radius bends (typically 5D and above) and can accommodate various tubing profiles, including square, rectangular, and oval sections. Roll bending is particularly effective for creating helical coils, spiral shapes, and large-diameter curves in architectural and industrial applications.
CNC Bending: Computer Numerical Control (CNC) bending machines integrate multiple bending techniques (such as rotary draw and roll bending) into a single, programmable platform. This advanced method offers high precision, repeatability, and the ability to produce complex multi-bend parts with minimal setup time. CNC bending is increasingly used in high-volume production environments and for creating intricate tubular assemblies in aerospace and automotive industries.
Heat Induction Bending: This specialized technique employs localized induction heating to precisely soften the bend zone before forming. An induction coil heats the tube to just below its recrystallization temperature (typically 1800-2000°F for austenitic stainless steels), followed by immediate bending using a forming die. This method allows for tight radius bends (down to 3D) with minimal wall thinning and ovality, even in thick-walled or large-diameter tubing. Heat induction bending is particularly valuable for heavy-wall pipes in oil & gas, power generation, and chemical processing industries.
When selecting a bending technique, engineers must consider factors such as material grade (e.g., 304, 316L), tube dimensions, bend radius, production volume, and end-use requirements. Each method has its unique benefits and limitations in terms of achievable geometries, surface finish, and cost-effectiveness. Employing the most suitable technique, often in combination with proper tooling and lubrication, ensures successful bending of stainless steel tubing while maintaining its mechanical properties and corrosion resistance.
When bending stainless steel tubing, selecting the appropriate equipment and tools is crucial for achieving precise, consistent results while maintaining the material’s integrity. This section outlines two primary types of tube benders: manual and hydraulic, highlighting their key features, capabilities, and considerations for use in stainless steel fabrication.
Manual Tube Benders
Manual tube benders are precision instruments that offer a balance of accuracy, affordability, and versatility for stainless steel tubing applications. These hand-operated devices utilize mechanical leverage to exert controlled force on the tubing, shaping it to the desired bend angle and radius. Key features and considerations include:
However, manual tube benders have limitations, particularly when working with larger diameter (typically >1.5 inches OD) or thick-walled stainless steel tubes (wall thickness >0.065 inches). These applications may require significant physical effort and can lead to operator fatigue, potentially compromising bend quality or production efficiency.
Hydraulic Tube Benders
Hydraulic tube benders leverage fluid power systems to generate the substantial forces required for bending stainless steel tubing, offering enhanced capabilities for larger-scale or high-volume production environments. Key advantages and considerations include:
While hydraulic tube benders offer superior capabilities for demanding stainless steel applications, they typically require a higher initial investment and ongoing maintenance to ensure proper hydraulic system function and calibration. Additionally, operator training is often more extensive to fully leverage the advanced features and ensure safe operation.
The selection between manual and hydraulic tube benders for stainless steel applications should consider factors such as production volume, tube specifications (diameter, wall thickness, and alloy grade), required bend complexity, and overall project economics. Both types of equipment, when properly selected and operated, can deliver high-quality bends in stainless steel tubing, contributing to the fabrication of durable, corrosion-resistant components across various industries.
Industrial stainless steel tubing is widely utilized in various fluid handling systems due to its excellent corrosion resistance, making it a preferred choice in highly corrosive environments. However, bending stainless steel tubing for corrosive liquid applications requires careful consideration of several factors to ensure structural integrity and optimal performance.
Bending stainless steel tubing is a complex process that demands thorough understanding and expertise. Different grades and compositions of stainless steel exhibit varying levels of ductility, yield strength, and work-hardening characteristics, which significantly influence their bending behavior. Key considerations include:
The selection of an appropriate bending radius is crucial to prevent cracking and maintain the tubing’s structural integrity. For longitudinal bending (along the grain direction), a larger bending radius is typically required to mitigate the risk of cracking. Conversely, transverse bending (across the grain) often allows for tighter internal radii without compromising the outer surface integrity, due to the material’s anisotropic properties.
When bending thick-walled stainless steel tubing, localized preheating of the bend area can significantly enhance formability. This technique reduces the required bending force and minimizes the risk of cracking by temporarily increasing the material’s ductility. The preheating temperature and duration must be carefully controlled to avoid adverse effects on the material’s microstructure and corrosion resistance.
Stainless steel tubing exhibits significant springback due to its high yield strength and elastic recovery. The degree of springback is directly proportional to the material’s yield strength and the bend radius. To achieve the desired final bend angle, overbending is necessary. The required overbend angle increases with larger bend radii and higher yield strength materials. In extreme cases, stress-relief heat treatment may be necessary to stabilize the final shape.
Hot forming of stainless steel tubing can mitigate issues such as strain hardening, cracking, and deformation by altering the material’s microstructure at elevated temperatures. While this method facilitates easier bending, especially for large diameter or thick-walled tubing, it presents challenges:
Hot forming should be employed judiciously, with careful control of temperature, atmosphere, and cooling rates to maintain the desired material properties and surface finish.
The minimum internal bend radius for stainless steel tubing is a critical parameter that depends on several factors:
A practical approach to estimating the minimum bend radius involves using 50% of the material’s elongation value provided by the supplier as a starting point. However, this should be validated through testing and adjusted based on specific application requirements and quality standards.
Additional Considerations:
The 304 stainless steel round pipe is a hollow, round stainless steel pipe that is widely used in various building decoration applications such as fences, stair handrails, and courtyard doors.
The bending process must be smooth, accurate in terms of angle, and free of cracks.
Next, I will explain the bending technology for 304 stainless steel round pipes.
1. Hot bending method:
First, in preparation for bending the 304 stainless steel round pipe, secure the arc car’s rollers onto an iron plate based on the outer diameter of the pipe. Fill the pipe with sand and plug both of its ends with wooden plugs. Then, preheat the section of the pipe that is going to be bent with a flame.
2. Cold bending method:
Before using the pipe bending machine, it is important to thoroughly test the machine. To avoid cracking, there should be a gap equal to the thickness of the plate at the bottom and a bending notch with a depth of 8 times the thickness of the plate should be selected.
See also:
1. During machining, the friction between the workpiece and the tool will generate high heat, and the tool is easy to wear.
Therefore, the requirements for tool materials are more strict, requiring wear resistance and high temperature resistance.
And must meet the requirements of sharp edges.
2. The bending process is slow and requires a large force, leading to low production efficiency.
In addition, due to the high heating capacity, sulfur chloride is generally used as a good cooling oil, and the effect is better.
Therefore, it is important for workers who process 304 stainless steel round pipes to have a good level of experience and technical skill.
1. Bend:
The general requirements for 304 stainless steel round pipe bending radius (R) are that it should be at least 1.5 to 2 times the diameter.
If the bending radius (R) is too small, the bend will be flat.
The bending radius (R) of the same stainless steel pipe should be consistent in order to meet the consistency of the wheel die.
The straight edge length of the bend must be at least 2 times the diameter, otherwise the bend die cannot be clamped smoothly.
2. Process hole:
Process holes shall be reserved for 304 stainless steel round pipe welds to better discharge welding slag generated during welding.
3. Processing performance:
First, consider the machinability of 304 stainless steel round pipes.
Stainless steel has high plasticity, toughness, cutting energy consumption, cutting temperature, low thermal conductivity, poor heat dissipation, and can easily cause tool temperature rise.
These factors can affect the bonding and weldability of stainless steel and cause blockages during the cutting process, leading to a reduction in the surface finish of the stainless steel pipes.
The bend radius is a critical parameter in stainless steel tubing quality, representing the curvature of the tube bend. Determining the optimal bend radius involves considering the tube’s diameter, wall thickness, material properties, and intended application. A well-chosen bend radius is essential to maintain structural integrity, prevent material failure, and ensure optimal performance of the tubing system.
Diameter to Radius Ratio: While the 2:1 diameter-to-radius ratio is a common guideline, advanced manufacturing techniques and material innovations now allow for tighter bends in certain applications. For instance, mandrel bending can achieve ratios as low as 1:1 for some stainless steel grades, though this requires precise tooling and process control.
Material Properties: The bendability of stainless steel is influenced by its microstructure, work hardening rate, and ductility. Austenitic grades like 304 and 316 generally offer superior bendability due to their face-centered cubic structure, while ferritic and martensitic grades may require larger bend radii or intermediate annealing steps to prevent cracking.
Springback is an elastic recovery phenomenon inherent in metal forming processes, where the bent tube partially returns to its original shape upon release of bending forces. This effect can lead to dimensional inaccuracies and compromised fit in complex assemblies if not properly managed.
Bending Process: The magnitude of springback is influenced by various factors:
Corrective Measures: Advanced springback compensation techniques include:
Inspection Methods:
To ensure bend quality meets specifications, employ a combination of visual, mechanical, and non-destructive testing methods:
Common Bending Issues
When bending stainless steel tubing, identifying and addressing potential issues is critical to ensure product integrity and performance. Common bending problems include:
Kinking: Occurs when the tubing collapses or folds at the bend point, typically due to inadequate tooling setup or improper mandrel selection. Kinking can compromise structural integrity, leading to potential leaks, stress concentrations, or premature failure.
Ovality: Manifests as a loss of circular cross-section, resulting in an oval profile. This issue often stems from improper die selection, insufficient back pressure, or excessive bending force. Ovality can affect fluid flow characteristics and fitting compatibility.
Wrinkling: Characterized by small folds or waves along the inner radius of the bend. Typically caused by insufficient mandrel support, improper wiper die setup, or excessive compressive forces during bending. Wrinkles can create turbulence in fluid systems and act as stress risers.
Springback: A natural phenomenon where the bent tube partially returns to its original shape due to elastic recovery. The degree of springback varies based on material properties, bend radius, and wall thickness. Failure to account for springback can result in inaccurate bend angles and geometries.
Wall thinning: Occurs on the outer radius of the bend due to material stretching. Excessive wall thinning can compromise the tube’s pressure-bearing capacity and corrosion resistance.
Corrective Measures
To ensure the longevity, reliability, and optimal performance of bent stainless steel tubing, implement the following corrective measures:
Optimized tooling selection: Utilize material-specific tooling, including properly sized mandrels, wiper dies, and pressure dies. Consider using urethane-insert bending dies for improved surface finish and reduced marking.
Adequate mandrel support: Select mandrels with appropriate plug and ball configurations to provide internal support during bending. Use mandrel lubricant to reduce friction and prevent scoring of the inner surface.
Pressure die adjustment: Fine-tune pressure die settings to maintain consistent wall contact without excessive compression. Consider using a rotary-draw bending process for improved control over material flow.
Wiper die optimization: Adjust wiper die position and pressure to effectively prevent wrinkling while minimizing friction. Use high-quality wiper dies with appropriate relief angles for the specific material and bend radius.
Bend radius selection: Choose an appropriate bend radius (typically 3-4 times the tube outer diameter for stainless steel) to balance formability with performance requirements. Larger radii generally result in less wall thinning and reduced springback.
Material condition consideration: Account for the work-hardening characteristics of stainless steel. Annealed tubing may be preferable for tight radius bends, while strain-hardened tubing can offer better springback control for larger radii.
Lubrication strategy: Implement a comprehensive lubrication plan, using compatible lubricants for mandrels, wiper dies, and external tube surfaces to reduce friction and improve material flow.
Machine calibration and maintenance: Regularly calibrate bending equipment and maintain proper alignment of all components. Ensure consistent clamping pressure and smooth operation of moving parts.
Process parameter optimization: Fine-tune bending speed, back pressure, and boost pressure settings. Utilize computer-controlled bending machines for precise, repeatable results and the ability to compensate for material variations.
Quality control measures: Implement rigorous inspection protocols, including the use of coordinate measuring machines (CMMs) or optical scanning systems to verify bend angles, ovality, and wall thickness consistency.
Industry Codes
Adherence to industry codes and standards is paramount when bending stainless steel tubing to ensure precision, reliability, and compliance. Key standards include:
ASME B31.1: Power Piping Code – Governs the design, fabrication, and installation of power piping systems, including specific requirements for bending stainless steel tubing.
ASME B31.3: Process Piping Code – Addresses process piping in refineries and chemical plants, detailing bending procedures and quality control for stainless steel tubing.
ASTM A269/A269M: Standard Specification for Seamless and Welded Austenitic Stainless Steel Tubing – Outlines material properties and tolerances crucial for bending operations.
AWS D18.1/D18.1M: Specification for Welding of Austenitic Stainless Steel Tube and Pipe Systems – Provides guidelines for welding bent tubing, ensuring integrity of the final assembly.
Compliance with these standards ensures structural integrity, performance reliability, and safety of bent stainless steel tubing in various applications.
Material Specifications
Material specifications are critical in determining the bending characteristics and final product performance. Key considerations include:
1. Stainless Steel Grade:
2. Tubing Dimensions:
3. Mechanical Properties:
4. Metallurgical Condition:
5. Surface Finish:
Selecting appropriate material specifications is crucial for achieving optimal bending results, meeting performance requirements, and ensuring longevity of the bent stainless steel tubing in its intended application. Engineers must carefully balance these factors against project requirements and manufacturing capabilities to achieve the best outcomes.