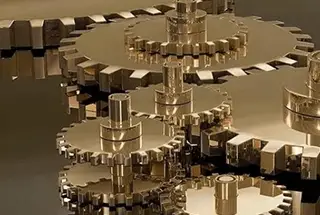
Have you ever wondered why some steel structures stand the test of time while others falter? This article explores the world of bolted and welded connections in steel structures, highlighting their unique advantages and disadvantages. By the end, you’ll understand how these connections impact the durability and cost-efficiency of steel constructions.
Bolted and welded connections represent the two predominant methods of assembly in modern steel structures and mechanical systems. This paper offers a comprehensive comparison of these connection techniques, analyzing their respective advantages, limitations, and optimal applications in the context of steel fabrication and construction.
The integrity and performance of steel structures fundamentally rely on the effectiveness of joining discrete components, such as plates and profiles, into a cohesive whole. The selection and execution of connection methods at these critical junctures significantly impact not only the structural quality but also the overall cost-efficiency and constructability of the project. Consequently, connection design plays a pivotal role in determining the success of steel structures across various industries.
Historically, steel structures employed a diverse range of joining techniques, including cotter pins, bolts, rivets, and welds. However, the evolution of materials science, engineering practices, and construction methodologies has led to the obsolescence of certain methods. Notably, cotter pin and rivet connections have been phased out in contemporary steel construction due to their limitations in strength, efficiency, and adaptability. As such, this analysis will focus exclusively on the two dominant connection types in modern steel fabrication: bolted and welded joints.
By examining these connection methods in detail, this paper aims to provide engineers, fabricators, and project managers with valuable insights to inform decision-making processes in steel structure design and assembly. The comparison will encompass factors such as structural performance, ease of installation, quality control, cost considerations, and suitability for different environmental and loading conditions.
Welded connections are formed through the fusion of a welding rod and the components to be joined, utilizing the heat generated by an electric arc. As the molten material cools and solidifies, it forms a weld seam that integrates the separate parts into a unified structure.
In modern steel construction, welded connections are the predominant joining method. Manual arc welding and automated (or semi-automated) submerged arc welding are the most widely employed techniques in the industry.
Advantages
Welded structures offer several benefits over bolted connections:
(1) Elimination of cross-section weakening: Welded joints do not require drilling, preserving the structural integrity of the components. The absence of additional connecting elements simplifies construction, resulting in labor and material savings. This cost-effectiveness is one of the most significant advantages of welded connections.
(2) Enhanced structural properties: Welded structures provide superior sealing, high rigidity, and excellent overall integrity. Complex geometries, such as Y- and T-shaped connections between steel pipes, are more readily achievable through welding than with bolted connections or alternative joining methods.
Disadvantages
Despite their benefits, welded joints present certain challenges:
(1) Thermal effects: The high temperatures involved in the welding process can alter material properties in the heat-affected zone (HAZ).
(2) Weld quality concerns: Weld seams may contain various defects, and the base metal adjacent to the weld can experience localized embrittlement. These issues can lead to stress concentrations and potential crack propagation within the structure.
(3) Structural rigidity implications: The high rigidity of welded structures can facilitate crack propagation throughout the entire assembly. This characteristic, combined with potential low-temperature brittleness, requires careful consideration in design and material selection.
(4) Residual stresses: Uneven cooling and shrinkage during the welding process can induce residual stresses within the structure. These internal stresses may cause premature localized yielding under load and reduce the critical buckling stress in compression members.
(5) Geometric distortion: Differential thermal expansion and contraction during welding can result in residual deformation, such as warping in flat steel plates.
To mitigate these challenges, it is crucial to implement preventive measures during the design, fabrication, and installation phases. Adherence to the national standard “Quality Acceptance Specification for Steel Structure Engineering” is essential for weld seam inspection and acceptance.
Ensuring high-quality welded connections requires a comprehensive approach, including:
By addressing these factors, the risk of brittle weld failures can be significantly reduced, leading to safer and more reliable welded steel structures.
Bolted connections unify components through the use of bolts, a type of fastener. There are two types of bolted connections: standard bolt connections and high-strength bolt connections.
Bolts utilized in steel structural connections are classified into two primary categories: standard bolts and high-strength bolts. Standard bolts typically feature hexagonal heads and are graded as A, B, and C, each with distinct characteristics and applications.
Grade C bolts are commonly manufactured from Q235 steel, utilizing hot-rolled round steel. These coarse bolts have relatively lenient requirements for bolt hole fabrication, making them widely adopted in standard bolt connections where precision is less critical.
In contrast, Grade A and B standard bolts are precision-engineered, demanding stricter manufacturing tolerances for both the bolt and the corresponding bolt hole. Despite their higher quality, standard bolts are generally installed using manual wrenches without specific pre-tension requirements.
High-strength bolts in steel structures carry a specialized meaning. Their installation involves purpose-designed wrenches that ensure a prescribed pre-tension in the bolt, resulting in a specified pre-pressure on the contact surface of the connected plates. This pre-tension is crucial for the structural integrity and load-bearing capacity of the connection.
To achieve the required pre-tension values, high-strength bolts are fabricated from high-strength steels. It’s important to note that while Grade A and B standard bolts may also be made from high-strength steel, they are still classified as standard bolts due to their installation method and lack of pre-tension requirements.
High-strength bolts are categorized into performance grades, with 8.8 and 10.9 being the most common in structural applications. These bolts are typically manufactured from medium-carbon steel or alloy steel, subjected to heat treatment processes (quenching and tempering) to enhance their mechanical properties.
The 8.8 grade high-strength bolts boast a minimum tensile strength (fub) of 800 N/mm², with a yield strength ratio of 0.8. The higher-grade 10.9 bolts offer even greater strength, with a minimum tensile strength of 1000 N/mm² and a yield strength ratio of 0.9. These superior mechanical properties make high-strength bolts ideal for critical structural connections where high load-bearing capacity and fatigue resistance are essential.
When selecting bolt types for steel structures, engineers must consider factors such as load requirements, connection type, installation method, and environmental conditions to ensure optimal performance and safety of the structural assembly.
Bolted connections are preferred in steel structures for their efficiency, simplicity, and lower skill requirements compared to welding. They rank second only to welded connections in usage frequency. Bolted connections are categorized into standard and high-strength bolt connections, each further classified based on stress conditions: shear-resistant, tension-resistant, and combined shear-and-tension-resistant connections.
Standard bolted connections typically employ coarse-threaded bolts (Grade C). Their shear resistance is determined by the bolt shaft’s shear strength and the hole wall’s compression resistance, while tensile resistance depends on the bolt’s axial pull capacity. These connections are primarily used in secondary components not directly subjected to dynamic loads, such as supports, rubbing strips, wall beams, small trusses, and removable structures. For on-site connections where bolts are under tension, coarse-threaded bolts are commonly utilized due to their superior tensile resistance.
Fine-threaded bolts (Grade A and B) are used in conventional connections requiring high shear resistance. However, due to their complex fabrication, stringent installation requirements (minimal clearance between bolt and hole), and higher cost, they are often replaced by high-strength bolt friction connections.
High-strength bolt bearing-type connections share material, preload, and installation requirements with friction-type connections. The key distinction lies in their ultimate bearing capacity mechanism. After overcoming friction, connected plates slip relative to each other, leading to bolt failure through shear and hole wall compression. This results in higher bearing capacity compared to friction connections, enabling material savings. However, the slip deformation post-friction limits their application to structures bearing static loads or indirectly subjected to dynamic loads. Surface preparation requirements are less stringent than friction connections, necessitating only the removal of oil and loose rust.
While bearing-type connections perform similarly to standard bolts, the preload on the bolt shaft and the use of high-strength steel enhance their performance beyond that of standard bolted connections. This makes them a valuable option in specific structural applications where higher load capacity is required without the complexity of friction-type connections.
Advantages of bolted connections:
Disadvantages of bolted connections:
Corrosion susceptibility: The interface between bolted components can trap moisture and debris, potentially accelerating corrosion if not properly sealed or protected.
Increased manufacturing complexity: They necessitate precise hole drilling in the plates, which must be accurately aligned during assembly. This increases manufacturing time, cost, and the potential for errors.
Higher precision requirements: Tight tolerances are essential for proper fit and load distribution, demanding more stringent quality control measures during fabrication and assembly.
Reduced cross-sectional area: Bolt holes weaken the effective cross-section of components, potentially compromising their load-bearing capacity and requiring compensation through increased material thickness or additional reinforcement.
Structural complexity: Connected parts often require overlapping or additional connection elements (e.g., gusset plates, angle steel), increasing the overall complexity of the structure and material consumption.
Maintenance considerations: Bolted connections may require periodic inspection and re-tightening due to potential loosening under dynamic loads or thermal cycling, necessitating ongoing maintenance protocols.
Stress concentration: Bolt holes can create stress concentration points, potentially leading to fatigue issues in structures subject to cyclic loading.