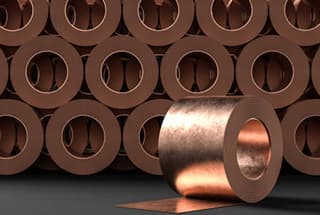
Have you ever considered the importance of material selection in engineering? In this blog post, we’ll explore the fascinating world of brass and its remarkable corrosion resistance properties. As an experienced mechanical engineer, I’ll share valuable insights into how brass withstands the test of time in various applications. Get ready to discover the science behind this alloy’s durability and learn how to leverage its benefits in your projects.
Brass, a Cu-Zn alloy with zinc as the main alloying element, gets its name from its yellow color.
Depending on the type and content of alloying elements added, brass can be categorized into three main types: single-phase brass, duplex brass, and special brass.
When the zinc content is below 36%, it forms a single-phase α solid solution, hence known as α brass. When the zinc content ranges between 36% and 45%, it becomes α+β duplex brass.
Brass with zinc content over 45% is impractical due to excessive brittleness from too much β phase. Special brasses are formulated by adding elements such as Sn, Mn, Al, Fe, Ni, Si, Pb, etc., to the Cu-Zn base.
Brass corrodes slowly in the atmosphere and also has a low corrosion rate in pure freshwater (0.0025-0.025mm/year). However, it corrodes somewhat faster in seawater (0.0075-0.1mm/year).
Fluorides have a minimal impact on brass corrosion, chlorides have a more significant effect, while iodides cause severe corrosion. In water containing gases like O2, CO2, H2S, SO2, NH3, etc., the corrosion rate of brass increases sharply.
It corrodes easily in mineral water, especially water containing Fe2(SO4)3. Brass undergoes severe corrosion in nitric and hydrochloric acids, corrodes more slowly in sulfuric acid, and is resistant to NaOH solutions. Brass has better impact corrosion resistance than pure copper.
Special brasses have better corrosion resistance than ordinary brass. Adding about 1% Sn to brass significantly reduces dezincification corrosion and improves its resistance to seawater. Incorporating about 2% Pb into brass enhances its wear resistance, thereby greatly reducing its corrosion rate in flowing seawater.
To prevent dezincification, small amounts of As, Sb, or P (0.02%-0.05%) can be added. Naval brass containing 0.5%-1.0% Mn has increased strength and excellent corrosion resistance. In brass containing 65% Cu and 55% Cu, replacing part of the Zn with 12%-18% Ni changes the color to silver-white, hence it is called nickel silver or German silver.
This alloy exhibits excellent corrosion resistance in salt, alkali, and non-oxidizing acids. The extensive substitution of Ni for Zn prevents dezincification. Besides these corrosion characteristics, brass also experiences two significant forms of corrosion: dezincification and stress corrosion cracking.
Factors influencing stress corrosion cracking in brass include the corrosive medium, stress, alloy composition, and microstructure. A specific alloy only undergoes corrosion cracking under certain media and specific stress conditions.
Brass under tensile stress can experience stress corrosion in all environments containing ammonia (or NH4+), as well as in the atmosphere, seawater, freshwater, high-temperature and high-pressure water, and steam. For example, the cracking of brass bullet casings during the rainy season in summer (also known as “season cracking”) is a typical example of stress corrosion cracking in brass.
Moreover, the morphology of brass stress corrosion cracking can be either intergranular or transgranular. In film-forming solutions, intergranular fractures primarily occur, while in non-film-forming solutions, transgranular fractures are more common.
The mechanism of brass stress corrosion cracking is generally believed to involve the formation of a brittle cuprous oxide film on the brass surface in film-forming solutions. This film fractures under stress and strain, leading to crack propagation to the base metal, which then halts due to slip, exposing the crack tip to the corrosive solution.
The process of intergranular penetration, film formation, brittle fracture, and crack propagation repeats, ultimately resulting in a stepped fracture surface. In non-film-forming solutions, stress causes preferential dissolution of brass surface dislocations, leading to crack propagation along the path of highest dislocation density, causing fracture.
In brass with lower zinc content, dislocations are mainly cellular, and grain boundaries have the highest dislocation density, leading to intergranular fractures.
In high-zinc brass, dislocations are mainly planar, and stacking faults are the areas of highest dislocation density, leading to transgranular fractures.
Additionally, the congregation of zinc atoms at dislocations under stress increases the reactivity at these sites, thus increasing the crack propagation rate with higher zinc content.
Experimental studies show that in atmospheric conditions, industrial atmospheres most readily cause stress corrosion cracking in brass, with the shortest fracture life, followed by rural atmospheres; marine atmospheres have the least effect.
These differences in atmospheric environments are due to variations in SO2 content (highest in industrial atmospheres, lower in rural atmospheres, and almost non-existent in marine atmospheres).
In summary, the substances that mainly cause stress corrosion cracking in brass are ammonia and its derivatives, or sulfides. The effect of ammonia is well recognized, while the role of sulfides is less clear. Additionally, steam, oxygen, SO2, CO2, CN- have an accelerating effect on stress corrosion.
Tensile stress is a necessary condition for the occurrence of stress corrosion cracking in brass. The greater the tensile stress, the higher the sensitivity to stress corrosion cracking.
Eliminating residual tensile stress through low-temperature tempering can prevent stress corrosion cracking in brass.
The higher the zinc content in brass, the greater its sensitivity to stress corrosion cracking. The specific zinc content below which stress corrosion does not occur depends on the nature of the medium.
For instance, brass with less than 20% zinc content generally does not experience stress corrosion in natural environments, but low-zinc brass can undergo stress corrosion cracking in ammonia water.
The effects of other alloying elements on stress corrosion are as follows:
Silicon effectively prevents stress corrosion cracking in α brass. Si and Mn improve the resistance of α+β and β brass to stress corrosion. Under ammonia atmospheres, elements like Si, As, Ce, Mg improve the stress corrosion resistance of α brass.
In atmospheric conditions, Si, Ce, Mg, etc., enhance stress corrosion resistance. Exposure tests in industrial atmospheres indicate that adding Al, Ni, and Sn to Cu-Zn alloys reduces their tendency to undergo stress corrosion cracking.