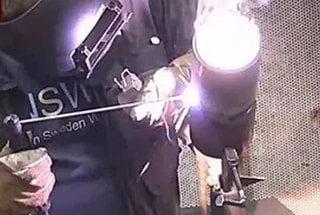
How can welding carbon steel be both a common practice and a complex challenge? This guide explores the intricate world of carbon steel welding, covering types of carbon steel, their weldability, and specific techniques to ensure strong, reliable joints. Readers will learn about the differences in welding low, medium, and high carbon steel, and the necessary precautions to avoid common welding defects. Dive in to understand the crucial steps and best practices for successful carbon steel welding.
1. What is Carbon Steel?
Steel is classified into two main categories based on its chemical composition: carbon steel and alloy steel. Carbon steel is further subdivided into three types according to its carbon content:
Low carbon steel, also known as mild steel, contains less than 0.25% carbon. It is characterized by:
Applications include:
Some low carbon steels undergo carburizing or other surface hardening treatments to enhance wear resistance for specific mechanical applications.
2. Medium Carbon Steel
Medium carbon steel, with 0.25% to 0.60% carbon content, offers:
Key characteristics:
Applications:
3. High Carbon Steel
High carbon steel, often referred to as tool steel, contains 0.60% to 1.70% carbon. It is characterized by:
Applications based on carbon content:
High carbon steels are typically heat-treated (quenched and tempered) to achieve optimal mechanical properties for their intended applications.
The weldability of steel is primarily influenced by its chemical composition, with carbon content being the most critical factor. While other alloying elements in steel can affect welding, their impact is generally less significant compared to carbon.
Low carbon steel (typically <0.25% C) exhibits excellent weldability and often requires minimal special processing. However, when working with thick plates (>25 mm), low ambient temperatures (<5°C), or applications with high mechanical demands, it is advisable to use basic electrodes and implement adequate preheating (150-200°C). For low carbon steels with carbon and sulfur content near their upper limits (C ~0.25%, S >0.05%), additional precautions are necessary:
Medium carbon steel (0.25-0.60% C) is more susceptible to cold cracking during welding. As carbon content increases, the hardenability of the HAZ rises, leading to a higher likelihood of cold cracking and reduced weldability. The elevated carbon levels in the base material also increase the carbon content in the weld metal, which, combined with any sulfur present, can promote hot cracking. To mitigate these issues when welding medium carbon steel:
High carbon steel (>0.60% C) presents the most significant welding challenges due to its high carbon content. During welding, substantial thermal stresses develop, the HAZ becomes highly susceptible to hardening and cold cracking, and the weld metal is prone to hot cracking. Consequently, high carbon steel has the poorest weldability among the three categories. Its use in welded structures is generally avoided, limiting applications to repair welding or hardfacing of wear-resistant components. When welding high carbon steel is necessary:
Medium carbon steel refers to carbon steel with a carbon content of 0.25% to 0.60%, which includes high-quality carbon structural steel grades such as 30, 35, 45, 50, 55 and cast carbon steel grades such as ZG230-450, ZG270-500, ZG310-570, and ZG340-640.
Due to the higher carbon content in medium carbon steel compared to low carbon steel, its weldability is inferior. When the mass fraction of carbon is close to 0.30% and the manganese content is not high, the weldability is still good, but as the carbon content increases, the weldability gradually worsens.
When the mass fraction of carbon reaches about 0.50%, the weldability significantly worsens.
The problems that can occur when welding medium carbon steel are as follows:
Due to the high carbon content in the steel, the heat-affected zone can easily produce hard and brittle martensite structure during welding, thereby leading to cold cracks.
If improper welding materials are used or the welding process is not rightly formulated, cold cracks can also easily occur in the weld.
During welding, the high carbon parent material will melt and introduce carbon into the weld, thereby increasing the carbon content in the welding. Carbon can intensify the effect of sulfur and phosphorus in metals to cause hot cracks.
Therefore, when welding medium carbon steel, hot cracks can easily occur in the weld. This is especially true when the sulfur and phosphorus content in the parent material or welding material are not strictly controlled, making hot cracks more likely to occur.
Additionally, the high carbon content in the steel can also increase the tendency of the weld to produce CO gas pores.
Due to the propensity of medium carbon steel to form defects such as cold and hot cracks when welded, special technical measures need to be implemented to ensure successful welding.
Various arc welding methods can be employed for medium carbon steel welding. As medium carbon steel is commonly used in machine part production rather than large-scale welding structures, shielded metal arc welding is most frequently utilized.
To prevent the formation of cold and hot cracks in the weld, low-hydrogen electrodes are typically used in shielded metal arc welding. These electrodes not only maintain a low hydrogen content in the weld but also exhibit desulfurizing and dephosphorizing effects, enhancing the plasticity and toughness of the weld.
When the steel has a lower carbon content and the joint has less rigidity, rutile or basic electrodes may be used. However, rigorous technical measures should be implemented, such as minimizing the fusion ratio, strictly preheating the workpiece, and controlling the interlayer temperature.
If preheating is not possible, austenitic stainless steel electrodes, such as E308L-16 (A102), E308L-15 (A107), E309-16 (A302), E309-15 (A307), E310-16 (A402), E310-15 (A407), can be used.
Preheating is the most effective technique to prevent cracking when welding medium carbon steel. Preheating not only reduces the cooling rate of the joint, preventing the formation of martensite but also reduces welding stress and expedites hydrogen diffusion.
In most circumstances, preheating and maintaining the interlayer temperature are necessary.
The selection of preheating and interlayer temperatures depends on the carbon equivalent of the steel, the thickness of the base metal, the rigidity of the structure, and the type of electrode.
The preheating temperature can be determined through welding tests, or through the empirical formula T0=550(C-0.12)+0.4δ. In this formula, T0 represents the preheating temperature (℃), C represents the mass fraction of carbon in the base metal being welded (%), and δ represents the thickness of the steel plate (mm).
The preheating and interlayer temperatures for welding 30, 35, and 45 steel can be referenced in Table 1.
Table 1 Preheating temperature and post weld tempering temperature for carbon steel welding
Steel Grade | Weldment thickness /mm | Operation process | Welding rod category | Note | |
Preheating interlayer temperature /℃ | Stress relief tempering temperature /℃ | ||||
30 | -25 | >50 | 600-650 | Non low hydrogen type welding rod | 1. The heating range on both sides of the groove for local preheating is 150-200mm 2. During the welding process, hammering can be used to reduce welding residual stress. |
Low hydrogen type welding rod | |||||
35 | 25-50 | >100 | Low hydrogen type | ||
>150 | Non low hydrogen type | ||||
50-100 | >150 | Low hydrogen type | |||
45 | -100 | >200 | Low hydrogen type |
The workpiece should ideally have a U or V-shaped groove to reduce the proportion of base metal melting into the weld. If repairing defects in castings, the dug-out groove should have a smooth exterior to minimize the amount of base metal that melts into the weld.
Direct current reverse polarity power supply should be used for welding. For multi-layer welding, small diameter electrodes, low current, and slow welding speed should be used since the proportion of base metal that melts into the first layer of the weld can reach up to 30%.
After welding, the workpiece should ideally undergo stress-relief heat treatment immediately. This is especially important for large thickness weldments, highly rigid structures, and weldments operating under dynamic or impact loads.
The temperature for stress-relief annealing is generally between 600 and 650 degrees Celsius.
If stress-relief heat treatment cannot be conducted immediately after welding, post-heating should be performed, which involves heating slightly above the preheat temperature, with a holding time of approximately 1 hour per 10mm of thickness.
(I) 35 Steel and ZG270-500 Cast Carbon Steel
The mass fraction of carbon in 35 steel is 0.32% to 0.39%, and that in ZG270-500 cast carbon steel is 0.31% to 0.40%. The carbon equivalent is about 0.45%, hence the weld-ability of this type of steel is acceptable.
However, in the heat-affected zone during welding, a hard and brittle martensitic structure may form, which tends to crack. Therefore, certain technical measures should be taken when welding this type of steel.
When using electrode arc welding, if a weld seam of equal strength to the parent material is required, E5016 (J506) or E5015 (J507) welding rods can be used. If a weld seam of equal strength to the parent material is not required, E4316 (J426), E4315 (J427), E4303 (J422), E4310 (J423) etc. welding rods can be selected.
For submerged arc welding, HJ430 or HJ431 fluxes and H08MnA or H10Mn2 wires can be selected.
For slag welding, HJ430, HJ431, HJ360 fluxes and H10Mn2, H08Mn2Si, H08Mn2SiA wires can be selected.
When welding 35 steel and ZG270-500 cast steel, the typical preheating temperature and interlayer temperature for the welded parts are around 150℃. If the rigidity of the welded parts is relatively large, the preheating temperature and interlayer temperature should be increased to 200-250℃.
The heating range for local preheating is 150-200mm on both sides of the groove.
For welded parts with high thickness, high rigidity, or working under dynamic or impact loads, stress relief annealing should be performed immediately after welding. The annealing temperature is generally 600-650℃.
For general thickness welded parts, post-heating can be used to allow the diffusion of hydrogen to escape.
The post-heating temperature is generally 200-350℃, and the holding time is 2-6 hours, depending on the thickness of the welded parts.
(II) 45 Steel and ZG310-570 Cast Carbon Steel
The mass fraction of carbon in 45 steel is 0.42% to 0.5%, and that in ZG310-570 cast steel is 0.41% to 0.50%. The carbon equivalent is about 0.56%. This steel has a greater tendency to harden and is prone to cracking, making its weld-ability relatively poor.
For electrode arc welding, low hydrogen welding rods should be chosen. If a weld seam of equal strength to the parent material is required, E5516-G (J556) or E5515-G (J557) welding rods can be used.
If a weld seam of equal strength to the parent material is not required, E4316 (J426), E4315 (J427), E5016 (J506), E5015 (J507), E4303 (J422), E4301 (J423) etc. welding rods can be selected.
For submerged arc welding, HJ350 or SJ101 fluxes and H08MnMoA wires can be selected.
When welding 45 steel and ZG310-570 cast carbon steel, a smaller welding current should be chosen to reduce the fusion ratio of the weld seam and decrease the amount of carbon transitioning from the parent material to the weld seam.
For welding this type of steel, it is best to preheat the entire piece to a temperature above 200℃.
For T-joints, because they have more heat dissipation directions than butt joints, the cooling speed of the welded joint will increase, raising the tendency to produce cold cracks.
Therefore, the preheating temperature should be appropriately increased to 250-400℃, depending on the thickness of the welded parts.
The interlayer temperature should not be lower than the preheating temperature.
After welding, the welded parts should immediately undergo stress relief annealing. The annealing temperature is 600-650℃.