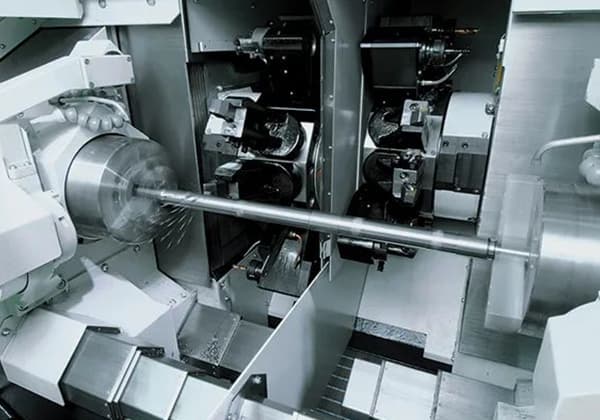
Have you ever thought about how tiny metal chips impact the machining process? In metal cutting, chip formation plays a crucial role in efficiency and safety. From spiral coils to fragmented pieces, each type of chip can affect the quality and safety of your work. This article explores the factors that influence chip formation, offering insights into optimizing your metal cutting operations for better control and improved results. Dive in to learn how to manage chip formation and enhance your machining process.
In metal cutting operations, some chips form into spiral coils, breaking off automatically at a certain length; others fold into C-shaped or S-shaped fragments.
Some take the form of spring-like spirals, while others fragment into needle-like or small pieces, scattering everywhere, compromising safety.
Ribbon-like chips can wind around the tools and workpieces, posing accident risks. Poor chip control can impede normal production.
The alloy elements, hardness, and heat treatment status of the workpiece material impact the thickness and curling of the chips. Soft steel forms thicker chips than hard steel, and hard steel is less prone to curling.
Chips that don’t curl easily are thin, but soft steel also resists curling when chip thickness is too large. The outer shape of the workpiece is another significant factor.
Appropriate geometric parameters of the tool’s cutting zone is the most common method to enhance the control of chip formation and reliability of chip breaking.
The rake angle is inversely proportional to chip thickness and has an optimal value for different materials being machined; the main clearance angle directly influences chip thickness and width, with a large angle facilitating chip breaking.
The radius of the tool tip arc relates to chip thickness, width, and the direction of chip flow.
Fine machining is suitable for a smaller radius, while rough machining is better suited for a larger radius.
The width of the chip breaking groove is proportionally selected according to the feed rate – a narrow one for a small feed rate and a wider one for a large feed rate.
The depth of the chip breaking groove is inversely selected according to the feed rate – a deep one for a small feed rate and a shallow one for a large feed rate.
The three elements of cutting parameters define the range of chip removal.
Feed rate and back cutting depth have the most significant impact on chip removal, while cutting speed within the standard range has the least effect.
Feed rate is directly proportional to chip thickness; back cutting depth is directly proportional to chip width; chip speed is inversely proportional to chip thickness. Increasing cutting speed narrows the effective range for chip removal.
Modern CNC machine tools employ NC editing functions to periodically adjust the feed rate, achieving what’s typically referred to as “program-controlled chip removal.
This method is highly reliable for chip removal but is less economical in terms of cutting. It’s commonly used in operations where chip removal is challenging, such as turning end faces and deep grooving.
The addition of cutting fluid broadens the effective chip removal range, particularly noticeable when small feed rates easily cause chip curling.
Utilizing high-pressure cutting fluids for chip breaking and removal is an effective method in certain machining processes, such as deep hole drilling, where high-pressure cutting fluids can expel chips from the cutting area.
The process of forming strip-like chips can be divided into three stages:
1. Basic Deformation Stage:
This is the phase where the metal layer being cut starts to interact with the cutting edge of the tool, leading to the detachment of the chip from the workpiece material.
2. Curling Deformation Stage:
This includes upward curling, sideways curling, and conical curling that involves both A and B directions.
3. Additional Deformation and Fracturing Stage.
Given the variations in workpiece materials and cutting conditions, a diverse range of chip shapes are produced during the cutting process.
The principal shapes of chips can be categorized into ribbon-like, segmented, granular, and fragmented types, as depicted in the figure.
1. Ribbon-like Chips
This is the most common type of chip. The inner surface is smooth, and the outer surface is fluffy.
When machining ductile metals, under conditions of small cutting thickness, high cutting speed, and larger tool rake angle, this type of chip often forms. The cutting process is balanced, with less fluctuation in cutting force, and the machined surface has a smaller roughness.
2. Segmental Chips
Also known as crushed chips. The outer surface is serrated, and the inner surface sometimes has cracks. This type of chip often forms when the cutting speed is lower, the cutting thickness is larger, and the tool rake angle is smaller.
3. Granular Chips
Also known as unit chips. In the process of chip formation, if the shear stress on the shear plane exceeds the fracture strength of the material, the crack extends to the entire surface, and the chip unit falls off from the cut material, forming granular chips, as shown in figure C.
The above three types of chips can only be obtained when processing ductile materials. Among them, the cutting process of ribbon-like chips is the most stable, and the fluctuation of cutting force of unit chips is the largest.
In production, ribbon-like chips are the most common, sometimes crushed chips are obtained, and unit chips are rarely seen.
If the conditions for crushed chips are changed, such as further reducing the tool rake angle, reducing the cutting speed, or increasing the cutting thickness, unit chips can be obtained. Conversely, ribbon-like chips can be obtained.
This indicates that the form of the chip can be transformed with the cutting conditions. Once its change rule is mastered, the deformation, form, and size of the chip can be controlled to achieve the purpose of chip curling and breaking.
4. Brittle Chip Formation
This type of chip formation occurs in brittle materials. The chips are irregular in shape, resulting in a rough and uneven machined surface.
In the cutting process, these chips undergo minimal deformation before fracturing, which differentiates them from chips formed by ductile materials.
The brittleness is primarily due to the applied stress surpassing the material’s tensile limit. Brittle and hard materials, such as high-silicon cast iron and white cast iron, often produce these chips, especially when the cutting thickness is substantial.
The cutting process is unstable, it can damage the tool, harm the machine tool, and roughen the already machined surface.
Therefore, it should be avoided in production. The methods to prevent this include reducing the cutting thickness, causing the chips to form in a needle-like or sheet-like shape, and appropriately increasing the cutting speed to enhance the ductility of the workpiece material.
The above are four typical types of chips, but the chips obtained in the processing site come in various shapes.
In modern cutting operations, the cutting speed and metal removal rate have reached high levels, creating harsh cutting conditions that often produce a substantial amount of “unacceptable” chips.
Appropriate measures should be taken in cutting processes to control chip curling, flow, and breakage, resulting in the formation of “acceptable” and well-shaped chips.
The most widely used chip control method in actual processing includes grinding a chip-breaking groove on the front face of the tool or using a clamp-style chip breaker.