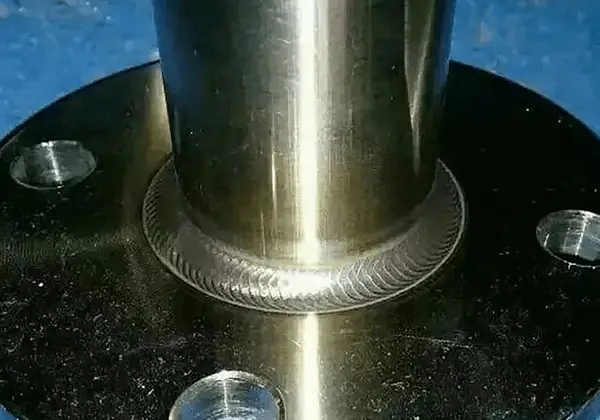
Ever wondered what makes stainless steel welding so resilient? In this article, we’ll explore the fascinating world of stainless steel welding rods, revealing how their unique compositions and properties make them essential for high-temperature and corrosive environments. You’ll learn which rods to use for specific materials and conditions, ensuring strong, durable welds every time.
Stainless steel welding rods are essential for joining corrosion-resistant or heat-resistant steels containing more than 10.5% chromium and less than 50% nickel. The selection of appropriate welding rods is critical and should be based on the specific stainless steel grade and operational conditions, including temperature and environmental factors.
For heat-resistant stainless steels operating at elevated temperatures, the primary focus is on ensuring weld crack resistance and maintaining high-temperature performance of the welded joint. In the case of austenitic heat-resistant steels such as 10Cr18Ni9Ti and Cr17Ni13, where the chromium-to-nickel ratio exceeds 1, austenite-ferrite stainless steel welding rods are typically recommended. For stabilized austenitic heat-resistant steels like Cr16Ni25Mo6 and Cr15Ni25W4Ti2, with a chromium-to-nickel ratio below 1, it’s crucial to match the weld metal composition to the base metal while increasing elements like molybdenum, tungsten, and manganese to enhance crack resistance.
When welding corrosion-resistant stainless steels exposed to various corrosive media, rod selection should be tailored to the specific environment and operating temperature. For applications above 300°C in highly corrosive settings, welding rods containing stabilizing elements such as titanium or niobium, or ultra-low carbon stainless steel rods are preferred. In environments with dilute sulfuric or hydrochloric acid, rods containing molybdenum or a combination of molybdenum and copper are typically chosen. For equipment operating at ambient temperatures in mildly corrosive conditions or where rust prevention is the primary concern, stainless steel welding rods without titanium or niobium are often sufficient.
When welding chromium stainless steels, such as martensitic 12Cr13 or ferritic 10Cr17Ti, chromium-nickel austenitic stainless steel welding rods are frequently employed to improve the ductility of the welded joint. This selection helps mitigate the potential for brittle fractures in these grades.
It’s important to note that the welding process, heat input, and post-weld heat treatment also play crucial roles in achieving optimal joint properties. Always consult the latest welding standards and manufacturer recommendations for specific applications, and consider conducting weld procedure qualification tests for critical components to ensure the desired mechanical properties and corrosion resistance are achieved.
According to the provisions of GB/T983-2012 “Stainless Steel Welding Rods,” the model number of stainless steel welding rods is divided based on the chemical composition of the deposited metal, coating type, welding position, and welding current type.
The method of compiling the model number is as follows:
a) The first part is represented by the letter “E” to indicate the welding rod.
b) The second part is the number following the letter “E,” indicating the classification of the deposited metal’s chemical composition. The letter “L” indicates a lower carbon content, and the letter “H” indicates a higher carbon content. If there are other special requirements for the chemical composition, it is represented by the elemental symbol placed after the number.
c) The third part is the first digit after the hyphen “-“, indicating the welding position, as shown in Table 2.
Table 2 Welding Position Code
Code | Welding position |
-1 | PA, PB, PD, PF |
-2 | PA, PB |
-4 | PA, PB, PD, PF, PG |
The explosive welding position is shown in GB/T16672, where PA=flat welding, PB=flat angle welding, PD=elevation angle welding, PF=upward vertical welding, PG=downward vertical welding
d) The fourth part is the last digit, indicating the coating type and current type, as shown in Table 3.
Table 3 Coating Type Codes
Code | Coating type | Current type |
5 | Alkalinity | DC |
6 | Rutile | AC and DC (a) |
7 | Titanic acid type | AC and DC (b) |
Model Example
Examples of complete electrode models in this standard are as follows:
E 308-1 6
Here are some specific selections of common austenitic, martensitic, and ferritic stainless steel welding rods:
1. Choosing austenitic stainless steel welding rods (see Table 1)
To ensure the weld metal of austenitic stainless steel maintains the same corrosion resistance and other properties as the base metal, the carbon content of the austenitic stainless steel welding rods should not be higher than that of the base metal.
Table 1 Selection of Commonly Used Austenitic Stainless Steel Welding Rods
Steel grade | Selection of welding rods | |
Grade | Model | |
022Cr19Ni10 06Cr18Ni9 | A002 A002 AA001G15 | E308L-16 E308L-17 E308L-15 |
06Cr19Ni9 | A101 A102 A102A A107 | E308-16 E308-17 E308-15 |
10Cr18Ni9 10Cr18Ni9Ti | A112 A132 A137 | — E347-16 |
06Cr18Ni10Ti 06Cr18Ni11Nb | A132 A137 | E347-16 E347-15 |
10Cr18Ni12Mo2Ti 06Cr18Ni12Mo2Ti | A202 A201 A207 | E316-16 E316-15 |
06Cr23Ni13 06Cr25Ni13 | A302 A301 A307 | E309-16 E309-15 |
10Cr25Ni18 06Cr25Ni20 | A402 A407 | E310-16 E310-15 |
2. Choosing martensitic stainless steel welding rods (see Table 2)
There are two types of rods used for welding martensitic stainless steel: chromium stainless steel welding rods and chromium-nickel austenitic stainless steel welding rods.
Table 2 Selection of Common Martensitic Stainless Steel Electrodes
Steel grade | Selection of welding rods | |
Grade | Model | |
12Cr13 20Cr13 | G202 G207 G217 | E410-16 E410-15 |
A102 A107 A302 A307 A402 A407 | E308-16 E308-15 E309-16 E309-15 E410-16 E410-15 E410-15 | |
14Cr17Ni2 | G302 G307 | E430-16 E430-15 |
A102 A107 A302 A307 A402 A407 | E308-16 E308-15 E309-16 E309-15 E410-16 E410-15 E410-15 |
3. Choosing ferritic stainless steel welding rods (see Table 3)
Due to the low toughness of the deposited metal from ferritic welding materials, combined with the difficulty of effectively transitioning added ferrite forming elements like Al, Ti into the weld pool, ferritic welding rods are not widely used.
Table 3 Selection of Ferritic Stainless Steel Welding Rods
Steel grade | Selection of welding rods | |
Grade | Model | |
022Cr12 06Cr13 | G202 G207 G217 | E410-16 E410-15 |
A302 A307 A402 A407 | E309-16 E309-15 E310-16 E310-15 | |
10Cr17 10Cr17Mo 022Cr17Mo 022Cr18Mo2 06Cr17Ti 10Cr17Ti | G302 G307 | E430-16 E430-15 |
A202 A207 A302 A307 A402 A407 | E316-16 E316-15 E309-16 E309-15 E309-15 E310-15 E310-16 E310-15 |
Grade | Standard model number (GB) | American standard model number (AWS) | Coating type | Welding current | Main applications |
G202 | E410-16 | E410-16 | Titanium-calcium type | AC/DC | Welding of surfacing 0Cr13, 1Cr13, and wear-resistant, corrosion-resistant surfaces. |
G207 | E410-15 | E410-15 | Low-hydrogen type | DC | Welding of surface build-up on 0Cr13, 1Cr13, and wear-resistant, corrosion-resistant materials. |
G217 | E410-15 | E410-15 | Low-hydrogen type | DC | Welding of surface overlay on 0Cr13, 1Cr13, and materials with wear and corrosion resistance. |
G302 | E430-16 | E430-16 | Titanium-calcium type | AC/DC | Welding of Cr17 stainless steel. |
G307 | E430-15 | E430-15 | Low-hydrogen type | DC | Welding of Cr17 stainless steel. |
A002 | E 308L -16 | E 308L -16 | Titanium-calcium type | AC/DC | Welding of ultra-low carbon Cr19Ni11 stainless steel and 0Cr19Ni10 stainless steel structures, such as synthetic fiber, fertilizer, petroleum, and other equipment. |
A012Si | Titanium-calcium type | AC/DC | Welding of ultra-low carbon C2 steel (OOCr17Ni15Si4Nb) used for resistance against concentrated nitric acid. | ||
A022 | E 316L -16 | E 316L -16 | Titanium-calcium type | AC/DC | Welding of urea and synthetic fiber equipment. |
A002N | E 316L -16 | E 316L -16 | Titanium-calcium type | AC/DC | Mainly used for welding 316LN stainless steel structures. |
A022Si | A | Titanium-calcium type | AC/DC | Used for welding 3RE60 lining plates or pipes in smelting equipment manufacturing. | |
A022MO | E317L-16 | E317L-16 | Titanium-calcium type | AC/DC | Used for welding ultra-low carbon 00Cr18Ni12Mo3 stainless steel, as well as for welding chromium stainless steels and composite steels that cannot undergo post-weld heat treatment, as well as dissimilar steels. |
A032 | E317MoCuL-16 | E317L-16 | Titanium-calcium type | AC/DC | Welding of structures made of ultra-low carbon stainless steel in equipment used for synthetic fibers and other applications, operating in dilute to medium concentration sulfuric acid environments. |
A042 | E309MoL-16 | E309MOL-16 | Titanium-calcium type | AC/DC | Welding of lining plates and overlay welding in urea synthesis towers, as well as welding of structures made of the same type of ultra-low carbon stainless steel. |
A052 | A | 1 | Titanium-calcium type | AC/DC | Welding of reactors, separators, and other equipment used in sulfuric acid, acetic acid, and phosphoric acid environments. |
A052Cu | A | Titanium-calcium type | AC/DC | Used for welding reactors, separators, and other equipment resistant to sulfuric acid, acetic acid, and phosphoric acid environments. | |
A062 | E 309L -16 | E 309L -16 | Titanium-calcium type | AC/DC | Welding of structures made of the same type of stainless steel, composite steel, and dissimilar steel used in synthetic fiber and petrochemical equipment. |
A072 | A | 1 | Titanium-calcium type | AC/DC | Used for welding of 00Cr25Ni20Nb steel, such as nuclear fuel equipment. |
A082 | A | 1 | Titanium-calcium type | AC/DC | Used for welding and repair welding of corrosion-resistant steels such as 00Cr17Ni15Si4Nb and 00Cr14Ni17Si4, which are resistant to concentrated nitric acid corrosion. |
A102 | E308-16 | E308-16 | Titanium-calcium type | AC/DC | Welding of corrosion-resistant 0Cr19Ni9, 0Cr19Ni11Ti stainless steel structures with working temperatures below 300°C. |
A102H | E308H-16 | E308H-16 | Titanium-calcium type | AC/DC | Welding of corrosion-resistant 0Cr19Ni9 stainless steel structures with working temperatures below 300°C. |
A107 | E308-15 | E308-15 | Low-hydrogen type | DC | Welding of corrosion-resistant 0Cr18Ni8 stainless steel structures with working temperatures below 300°C. |
A132 | E347-16 | E347-16 | Titanium-calcium type | AC/DC | Welding of critical titanium-stabilized 0Cr19Ni11Ti stainless steel. |
A137 | E347-15 | E347-15 | Low-hydrogen type | DC | Welding of critical titanium-stabilized 0Cr19Ni11Ti stainless steel. |
A157Mn | A | Low-hydrogen type | DC | Used for welding high-strength steel and dissimilar steel, such as H617 steel. | |
A146 | A | 1 | Low-hydrogen type | DC | Welding of critical 0Cr20Ni10Mn6 stainless steel structures. |
A202 | E316-16 | E316-16 | Titanium-calcium type | AC/DC | Welding of 0Cr17Ni12Mo2 stainless steel structures operating in organic and inorganic acid media. |
A207 | E316-15 | E316-15 | Low-hydrogen type | DC | Welding of 0Cr17Ni12Mo2 stainless steel structures operating in organic and inorganic acid media. |
A212 | E318-16 | E318-16 | Titanium-calcium type | AC/DC | Welding of critical 0Cr17Ni12Mo2 stainless steel equipment, such as urea and synthetic fiber equipment. |
A222 | E317MuCu-16 | 1 | Titanium-calcium type | AC/DC | Welding of stainless steel structures with the same type and copper content, such as 0Cr18Ni12Mo2Cu2. |
A232 | E318V-16 | 1 | Titanium-calcium type | AC/DC | Welding of general heat-resistant and corrosion-resistant stainless steel structures, such as 0Cr19Ni9 and 0Cr17Ni12Mo2. |
A237 | E318V-15 | 1 | Low-hydrogen type | DC | Welding of commonly used heat-resistant and corrosion-resistant stainless steel structures, such as 0Cr19Ni9 and 0Cr17Ni12Mo2. |
A242 | E317-16 | E317-16 | Titanium-calcium type | AC/DC | Welding of structures made of the same type of stainless steel. |
A302 | E309-16 | E309-16 | Titanium-calcium type | AC/DC | Welding of structures made of the same type of stainless steel, stainless steel liners, dissimilar steels (such as Cr19Ni9 with low carbon steel), as well as high-chromium steel, high-manganese steel, and so on. |
A307 | E309-15 | E309-15 | Low-hydrogen type | DC | Welding of structures made of the same type of stainless steel, dissimilar steels, high-chromium steel, high-manganese steel, and so on. |
A312 | E309Mo-16 | E309Mo-16 | Titanium-calcium type | AC/DC | Used for welding stainless steel containers resistant to sulfuric acid corrosion in the medium, as well as for welding stainless steel liners, composite steel plates, and dissimilar steels. |
A312SL | E309Mo-16 | E309Mo-16 | Titanium-calcium type | AC/DC | Used for welding aluminum-alloyed surface parts of Q235, 20g, Cr5Mo, and other steel materials, as well as for welding dissimilar steel materials. |
A316 | A | 1 | Titanium-calcium type | AC/DC | Used for welding of stainless steel, composite steel plates, and dissimilar steels resistant to corrosion in sulfuric acid media. |
A317 | E309Mo-15 | E309Mo-15 | Low-hydrogen type | DC | Used for welding of stainless steel, composite steel plates, and dissimilar steels resistant to corrosion in sulfuric acid media. |
A402 | E310-16 | E310-16 | Titanium-calcium type | AC/DC | Used for welding of heat-resistant stainless steel of the same type operating under high-temperature conditions, and can also be used for welding of hardenable chromium steel and dissimilar steels. |
A407 | E310-15 | E310-15 | Low-hydrogen type | DC | Used for welding of heat-resistant stainless steel of the same type, stainless steel liners, and can also be used for welding of hardenable chromium steel and dissimilar steels. |
A412 | E310Mo-16 | E310Mo-16 | Titanium-calcium type | AC/DC | Used for welding of heat-resistant stainless steel, stainless steel liners, and dissimilar steels operating under high-temperature conditions. It also exhibits excellent toughness when welding high-hardenability carbon steel and low-alloy steel. |
A422 | A | 1 | Titanium-calcium type | AC/DC | Used for welding and repair welding of Cr25Ni20Si2 austenitic heat-resistant steel drums on furnace coil rolling machines. |
A432 | E310H-16 | E310H-16 | Titanium-calcium type | AC/DC | Specifically used for welding HK40 heat-resistant steel. |
A462 | A | 1 | Titanium-calcium type | AC/DC | Used for welding furnace tubes (such as HK-40, HP-40, RC-1, RS-1, IN-80, etc.) that operate under high-temperature conditions. |
A502 | E16-25MoN-16 | 1 | Titanium-calcium type | AC/DC | Used for welding of dissimilar steels, low-alloy and medium-alloy steels in quenched and tempered condition, as well as structures with high strength. It is also suitable for welding of quenched and tempered 30CrMnSiA steel, as well as stainless steel, carbon steel, chromium steel, and dissimilar steels. |
A507 | E16-25MoN-15 | 1 | Low-hydrogen type | DC | Used for welding of dissimilar steels, low-alloy and medium-alloy steels in quenched and tempered condition, as well as structures with high strength. It is also suitable for welding of quenched and tempered 30CrMnSiA steel, as well as stainless steel and carbon steel. |
A512 | E 16-8-2 -16 | 1 | Titanium-calcium type | AC/DC | Mainly used for welding high-temperature and high-pressure stainless steel pipelines. |
A517 | A | Low-hydrogen type | DC | Used for welding rods of steel with equivalent resistance to sulfuric acid corrosion. | |
A607 | E330MoMnWNb-15 | 1 | Low-hydrogen type | DC | Used for welding of stainless steel materials of the same type that operate under high-temperature conditions of 850°C to 900°C, as well as for welding of collector pipes and expansion pipes in hydrogen conversion furnaces (such as Cr20Ni32 and Cr20Ni37 materials). |
A707 | A | 1 | Low-hydrogen type | DC | Used for welding of equipment used in acetic acid, vinyl, urea, and other applications. |
A717 | A | 1 | Low-hydrogen type | DC | Suitable for welding of 2Cr15Mn15Ni2N low-magnetic stainless steel components in electro-physical devices or for welding of dissimilar steel such as 1Cr18Ni11Ti. |
A802 | A | 1 | Titanium-calcium type | AC/DC | Welding of pipelines used in the manufacturing of synthetic rubber with a sulfuric acid concentration of 50% and specific working temperature and atmospheric pressure, as well as welding of Cr18Ni18Mo2Cu2Ti. |
A902 | E320-16 | E320-16 | Titanium-calcium type | AC/DC | Used for welding Carpenter 20Cb nickel alloy in corrosive media such as sulfuric acid, nitric acid, phosphoric acid, and oxidizing acids. |
Grade | AWS | Chemical composition of the deposited metal (%) | Mechanical properties of the deposited metal | Uses | |||||||||||||
C | Mn | Si | S | P | Cr | Ni | Mo | Cu | Others | R m (MPa) | A (%) | ||||||
E5MoV-15 | — | ≤0.12 0.074 | 0.5-0.9 0.68 | ≤0.50 0.42 | ≤0.030 0.010 | ≤0.030 0.019 | 4.5-6.0 5.3 | — | 0.40-0.70 0.55 | ≤0.5 0.052 | V : 0.10-0.35 0.25 | ≥540 625 (750℃×4h) | ≥14 20 (750℃×4h) | Used for welding of pearlitic heat-resistant steels such as Cr5MoV. | |||
E410-15 | E410-15 | ≤0.12 0.048 | ≤1.0 0.81 | ≤0.90 0.44 | ≤0.030 0.007 | ≤0.030 0.023 | 11.0-13.5 13.16 | ≤0.70 0.51 | ≤0.75 0.12 | ≤0.75 0.15 | — | ≥450 545 (750℃×1h) | ≥20 23 (750℃×1h) | Used for surface overlay welding of 0Cr13, 1Cr13 steel, and wear-resistant, corrosion-resistant steels. | |||
E410NiMo-15 | E410NiMo-15 | ≤0.06 0.030 | ≤1.0 0.71 | ≤0.90 0.26 | ≤0.030 0.006 | ≤0.030 0.016 | 11.0-12.5 12.15 | 4.0-5.0 4.39 | 0.40-0.70 0.45 | ≤0.75 0.17 | — | ≥760 890 (610℃×1h) | ≥15 17 (610℃×1h) | Used for welding of 0Cr13 stainless steel. | |||
E308-16 | E308-16 | ≤0.08 0.052 | 0.5-2.5 1.33 | ≤0.90 0.71 | ≤0.030 0.007 | ≤0.030 0.021 | 18.0-21.0 19.82 | 9.0-11.0 9.45 | ≤0.75 0.13 | ≤0.75 0.20 | — | ≥550 630 | ≥35 40 | Used for welding of 0Cr19Ni9 stainless steel structures with working temperatures below 300°C. | |||
E308-15 | E308-15 | ≤0.08 0.057 | 0.5-2.5 1.35 | ≤0.90 0.41 | ≤0.030 0.007 | ≤0.030 0.021 | 18.0-21.0 19.78 | 9.0-11.0 9.75 | ≤0.75 0.15 | ≤0.75 0.20 | — | ≥550 630 | ≥35 40 | Used for welding of 0Cr19Ni9 stainless steel structures with working temperatures below 300°C. | |||
E308H-16 | E308H-16 | 0.04-0.08 0.058 | 0.5-2.5 1.14 | ≤0.90 0.62 | ≤0.030 0.007 | ≤0.030 0.020 | 18.0-21.0 19.70 | 9.0-11.0 9.68 | ≤0.75 0.20 | ≤0.75 0.10 | — | ≥550 645 | ≥35 42 | Used for welding of 0Cr19Ni9 stainless steel structures with working temperatures below 300°C. | |||
E308L-16 | E308L-16 | ≤0.04 0.028 | 0.5-2.5 1.15 | ≤0.90 0.70 | ≤0.030 0.010 | ≤0.030 0.019 | 18.0-21.0 19.25 | 9.0-11.0 9.49 | ≤0.75 0.10 | ≤0.75 0.13 | — | ≥520 590 | ≥35 44 | Used for welding of ultra-low carbon 00Cr19Ni10 or 0Cr18Ni10Ti stainless steel. | |||
E308L-16W | E308L-16 | ≤0.04 0.029 | 0.5-2.5 2.14 | ≤0.90 0.53 | ≤0.030 0.010 | ≤0.030 0.019 | 18.0-21.0 19.25 | 9.0-11.0 10.2 | ≤0.75 0.10 | ≤0.75 0.13 | — | ≥520 590 | ≥35 44 -196℃ A KV 41(J) | Used for welding of ultra-low carbon 00Cr19Ni10 or 0Cr18Ni10Ti stainless steel, which exhibits good toughness at 196°C. It is suitable for welding LNG storage tanks and pipelines. |
Welding Characteristics and Electrode Selection of Austenitic Stainless Steel
Austenitic stainless steel is renowned for its exceptional weldability and widespread industrial applications. While it generally does not require specialized welding processes, understanding its unique characteristics is crucial for achieving optimal results. This paper provides a comprehensive analysis of potential welding defects in austenitic stainless steel, including hot cracking, intergranular corrosion, stress corrosion cracking, and various forms of weld joint embrittlement (low-temperature, sigma phase, and fusion line brittle fracture). Moreover, it offers practical prevention strategies for each of these issues.
Through a synthesis of theoretical principles and practical insights, this study delves into the intricacies of electrode selection for welding austenitic stainless steel. It explores how material composition, service conditions, and specific application requirements influence the choice of welding consumables. The paper emphasizes that achieving superior weld quality hinges on the synergy between appropriate process parameters and judicious electrode selection.
Stainless steel has become an indispensable material in high-performance industries such as aerospace, petrochemicals, advanced chemical processing, and nuclear power generation. Classification of stainless steels is typically based on either chemical composition (chromium vs. chromium-nickel) or microstructure (ferritic, martensitic, austenitic, and austenitic-ferritic duplex). Among these, austenitic stainless steel, often referred to as 18-8 stainless steel due to its typical chromium and nickel content, stands out for its superior corrosion resistance.
While austenitic stainless steel may have lower yield strength compared to some other grades, it compensates with excellent ductility, exceptional toughness, and superior weldability. These properties make it the material of choice for critical components in chemical processing equipment, pressure vessels, and various industrial applications where material integrity is paramount.
Despite its many advantages, the welding of austenitic stainless steel requires careful consideration. Improper welding techniques or unsuitable filler metal selection can lead to various defects that compromise the material’s performance. These may include sensitization, ferrite content imbalance, or intermetallic phase formation, all of which can detrimentally affect the corrosion resistance, mechanical properties, or service life of the welded structure.
By addressing these challenges through informed process design and material selection, engineers and welding professionals can fully leverage the capabilities of austenitic stainless steel, ensuring robust and reliable performance in demanding industrial environments.
Hot cracking is a defect that can readily occur when welding austenitic stainless steel, including longitudinal and transverse weld cracks, arc strike cracks, root cracks of the first run, and interlayer cracks in multi-layer welding. This is especially true for high-nickel austenitic stainless steels.
(1) Austenitic stainless steel has a large liquid-to-solid phase interval, resulting in a longer crystallization time and strong crystallographic orientation of single-phase austenite, leading to serious segregation of impurities.
(2) It has a small coefficient of thermal conductivity and a large linear expansion coefficient, resulting in large welding internal stresses (typically tensile stresses in the weld and heat-affected zone).
(3) Elements such as C, S, P, Ni in austenitic stainless steel can form low-melting eutectics in the weld pool. For example, Ni3S2 formed by S and Ni has a melting point of 645°C, while the Ni-Ni3S2 eutectic has a melting point of only 625°C.
(1) Use a duplex structure weld. Strive to make the weld metal an austenitic and ferritic duplex structure. Controlling the ferrite content below 3-5% can disrupt the direction of austenite columnar crystals and refine the grains. Also, ferrite can dissolve more impurities than austenite, reducing the segregation of low-melting eutectics at the austenite grain boundaries.
(2) Welding process measures. Quality alkaline-coated electrodes should be selected as far as possible, along with small line energy, small currents, quick non-oscillatory welding. When finishing, try to fill the crater and use argon arc welding for the first run to minimize welding stress and crater cracking.
(3) Control chemical composition. Strictly limit the content of impurities such as S, P in the weld to reduce low-melting eutectics.
Intergranular corrosion occurs between grains, causing loss of bonding strength between grains, with strength nearly completely disappearing. When subjected to stress, it will fracture along the grain boundaries.
According to the chromium depletion theory, when the weld and heat-affected zone are heated to the sensitization temperature of 450-850℃ (dangerous temperature zone), carbon, which is supersaturated, diffuses to the grain boundaries of the austenite due to the larger atomic radius of Cr and slower diffusion speed. It forms Cr23C6 with the chromium compound at the grain boundary, resulting in chromium-depleted grain boundaries, which are insufficient to resist corrosion.
(1) Control Carbon Content
Utilize low-carbon or ultra-low carbon (W(C) ≤ 0.03%) stainless steel welding materials such as A002.
(2) Add Stabilizers
Adding elements like Ti, Nb into the steel and welding materials, which have a stronger affinity with C than Cr, can combine with C to form stable carbides, thus preventing chromium depletion at the austenitic grain boundaries. Common stainless steel and welding materials contain Ti, Nb, such as 1Cr18Ni9Ti, 1Cr18Ni12MO2Ti steels, E347-15 electrodes, H0Cr19Ni9Ti welding wire, etc.
(3) Use a Duplex Structure
By introducing a certain amount of ferrite-forming elements such as Cr, Si, Al, Mo from welding wire or electrodes into the weld, a duplex structure of austenite + ferrite is formed in the weld. Since Cr diffuses faster in ferrite than in austenite, Cr diffuses towards the grain boundary in ferrite more quickly, reducing the chromium depletion at the austenite grain boundaries. The ferrite content in the weld metal is generally controlled to be 5% to 10%. If there is too much ferrite, the weld will become brittle.
(4) Rapid Cooling
Since austenitic stainless steel does not undergo hardening, the cooling rate of the welding joint can be increased during the welding process, for example, by placing a copper pad under the workpiece or directly cooling it with water.
In welding, small currents, high welding speeds, short arcs, and multi-pass welding can be used to reduce the dwell time of the weld joint in the dangerous temperature zone, avoiding the formation of chromium-depleted zones.
(5) Carry Out Solution Treatment or Homogenizing Heat Treatment
After welding, heat the weld joint to 1050-1100℃ to dissolve carbides back into the austenite, then quickly cool to form a stable single-phase austenitic structure.
Alternatively, carry out a homogenizing heat treatment, maintaining the temperature at 850-900℃ for 2 hours. At this time, Cr inside the austenite grains diffuses to the grain boundaries, and the Cr content at the grain boundaries reaches more than 12% again, thereby preventing intergranular corrosion.
Stress corrosion cracking is a form of destructive corrosion that occurs in metals under the combined action of stress and corrosive media. According to examples of stress corrosion failure in stainless steel equipment and components and experimental research, it can be assumed that under the joint action of certain static tensile stress and specific electrochemical media at certain temperatures, existing stainless steels may exhibit stress corrosion.
One of the key characteristics of stress corrosion is that the corrosive media and materials combination exhibits selectivity. Media likely to cause stress corrosion in austenitic stainless steel primarily includes hydrochloric acid and chloride-containing media, as well as sulfuric acid, nitric acid, hydroxides (alkalis), seawater, steam, H2S solution, concentrated NaHCO3+NH3+NaCl solution, and others.
Stress corrosion cracking is the delayed cracking phenomenon that occurs when a welded joint is subjected to tensile stress in a specific corrosive environment. Stress corrosion cracking in the weld joint of austenitic stainless steel is a serious failure mode, manifesting as brittle failure with no plastic deformation.
(1) Rational Processing and Assembly Procedures
Minimize cold deformation as much as possible, avoid forced assembly, and prevent various forms of damage (including assembly and arc burns) during assembly that can act as SCC crack sources and cause pitting corrosion.
(2) Rational Choice of Welding Material
Ensure a good match between the weld seam and the base material, and prevent any adverse structures such as grain coarsening and hard, brittle martensite.
(3) Suitable Welding Technique
Ensure the weld seam is well-formed and does not produce any stress concentration or pitting defects, such as undercutting. Adopt a reasonable welding sequence to reduce the level of residual welding stress. For instance, avoid cross-joints, change Y-shaped grooves to X-shaped grooves, appropriately reduce the groove angle, use short welding paths, and utilize low linear energy.
(4) Stress Relief Treatment
Implement post-weld heat treatment, such as full annealing or stress relief annealing. Use post-weld hammering or shot peening when heat treatment is challenging to implement.
(5) Production Management Measures
Control impurities in the media, such as O2, N2, H2O in liquid ammonia, H2S in liquefied petroleum gas, O2, Fe3+, Cr6+ in chloride solutions, etc. Implement anti-corrosion measures, such as coating, lining, or cathodic protection, and adding corrosion inhibitors.
After austenitic stainless steel welds have been heated at high temperatures for a certain period, a decrease in impact toughness occurs, known as embrittlement.
(1) Causes
The structure of duplex welds containing a large amount of ferrite phase (over 15%~20%) will experience a significant decrease in plasticity and toughness after heating at 350~500°C. Since the embrittlement rate is fastest at 475°C, this is called 475°C embrittlement.
For austenitic stainless steel weld joints, corrosion resistance or oxidation resistance is not always the most critical performance. When used at low temperatures, the plasticity and toughness of the weld metal become key properties.
To meet the requirements for low-temperature toughness, a single austenite structure is typically desired for the weld structure to avoid the presence of δ ferrite. The presence of δ ferrite always worsens low-temperature toughness, and the more it contains, the more severe the embrittlement.
(2) Preventive Measures
① While ensuring the crack resistance and corrosion resistance of the weld metal, the ferrite phase should be controlled at a lower level, around 5%.
② Welds that have undergone 475°C embrittlement can be eliminated by quenching at 900°C.
(1) Causes
When austenitic stainless steel weld joints are used for an extended period in the temperature range of 375~875°C, an FeCr intermetallic compound known as the σ phase is produced. The σ phase is hard and brittle (HRC>68).
The precipitation of the σ phase results in a sharp decrease in the impact toughness of the weld, a phenomenon known as σ phase embrittlement. The σ phase generally only appears in duplex structure welds; when the operating temperature exceeds 800~850°C, the σ phase will also precipitate in single-phase austenite welds.
(2) Preventive Measures
① Limit the content of ferrite in the weld metal (less than 15%); use superalloy welding materials, i.e., high-nickel welding materials, and strictly control the content of Cr, Mo, Ti, Nb, and other elements.
② Use a small specification to reduce the dwell time of the weld metal at high temperatures.
③ For already precipitated σ phase, perform solution treatment when conditions allow, to dissolve the σ phase into austenite.
④ Heat the weld joint to 1000~1050°C and then rapidly cool. The σ phase generally does not occur in 1Cr18Ni9Ti steel.
(1) Causes
When austenitic stainless steel is used at high temperatures for an extended period, brittle fracture can occur along the fusion line.
(2) Preventive Measures
Adding Mo to the steel can improve the steel’s ability to resist high-temperature brittle fracture.
From the above analysis, it can be seen that the correct choice of welding process measures or welding materials can prevent the occurrence of the above welding defects. Austenitic stainless steel has excellent weldability, and almost all welding methods can be used for welding austenitic stainless steel.
Among various welding methods, shielded metal arc welding (SMAW) is widely used due to its adaptability to various positions and different plate thicknesses. Next, let’s analyze the selection principles and methods of austenitic stainless steel welding rods for different purposes.
Stainless steel is mainly used for corrosion resistance, but it is also used for heat-resistant and low-temperature steels.
Therefore, when welding stainless steel, the performance of the welding rod must match the intended use of the stainless steel. The selection of stainless steel welding rods must be based on the base metal and working conditions, including operating temperature and contact media.
Table of different stainless steel grades and corresponding welding rod types and numbers.
Steel grade | Welding rod model | Welding rod grade | Nominal composition of the welding rod | Note |
0Cr18Ni11 | E308L-16 | A002 | 00Cr19Ni10 | |
0Cr19Ni11 | ||||
00Cr17Ni14Mo2 | Excellent heat resistance, corrosion resistance, and crack resistance | |||
00Cr18Ni5Mo3Si2 | E316L-16 | A022 | 00Cr18Ni12Mo2 | |
00Cr17Ni13Mo3 | ||||
00Cr18Ni14Mo2Cu2 | E316Cu1-16 | A032 | 00Cr19Ni13Mo2Cu | |
00Cr22Ni5Mo3N | E309Mo1-16 | A042 | 00Cr23Ni13Mo2 | |
Corrosion resistance of the weld to formic acid, acetic acid, and chloride ions | ||||
00Cr18Ni24Mo5Cu | E385-16 | A052 | 00Cr18Ni24Mo5 | |
0Cr19Ni9 | E308-16 | A102 | 0Cr19Ni10 | Titanium-calcium type coating |
1Cr18Ni9Ti | ||||
1Cr19Ni9 | E308-15 | A107 | 0Cr19Ni10 | Low-hydrogen type coating |
0Cr18Ni9 | ||||
0Cr18Ni9 | — | A122 | — | |
Possessing excellent resistance to intergranular corrosion | ||||
0Cr18Ni11Ti | E347-16 | A132 | 0Cr19Ni10Nb | |
0Cr18Ni11Nb | E347-15 | A137 | 0Cr19Ni10Nb | |
1Cr18Ni9Ti | ||||
0Cr17Ni12Mo2 | E316-16 | A202 | 0Cr18Ni12Mo2 | |
00Cr17Ni13Mo2Ti | ||||
1Cr18Ni12Mo2Ti | Having better resistance to intergranular corrosion compared to A202 | |||
00Cr17Ni13Mo2Ti | E316Nb-16 | A212 | 0Cr18Ni12Mo2Nb | |
0Cr18Ni12Mo2Cu2 | E316Cu-16 | A222 | 0Cr19Ni13Mo2Cu2 | Due to the presence of copper, it exhibits excellent acid resistance in sulfuric acid media. |
0Cr19Ni13Mo3 | With a high molybdenum content, it has excellent resistance to non-oxidizing acids and organic acids. | |||
00Cr17Ni13Mo3Ti | E317-16 | A242 | 0Cr19Ni13Mo3 | |
1Cr23Ni13 | E309-16 | A302 | 1Cr23Ni13 | Dissimilar steels, high-chromium steels, high-manganese steels, etc. |
00Cr18Ni5Mo3Si2 | ||||
00Cr18Ni5Mo3Si2 | E309Mo-16 | A312 | 1Cr23Ni13Mo2 | |
Used for welding of high-hardenability chromium steel and dissimilar steel. | ||||
1Cr25Ni20 | E310-16 | A402 | 2Cr26Ni21 | |
1Cr18Ni9Ti | E310-15 | A407 | Low-hydrogen type coating | |
Cr16Ni25Mo6 | E16-25MoN-16 | A502 | ||
Cr16Ni25Mo6 | E16-25MoN-15 | A507 |
(I) Key Point One
Generally, the selection of welding rods can refer to the material of the base metal, choosing welding rods that have the same or similar composition as the base metal. For example, A102 corresponds to 0Cr18Ni9, A137 corresponds to 1Cr18Ni9Ti.
(II) Key Point Two
Since the carbon content greatly impacts the corrosion resistance of stainless steel, it’s generally recommended to select stainless steel welding rods where the deposited metal contains a lower amount of carbon than the base metal. For instance, an A022 welding rod must be chosen for 316L.
(III) Key Point Three
The weld metal of austenitic stainless steel should ensure mechanical properties. This can be verified through a welding process evaluation.
(IV) Key Point Four (Austenitic Heat-Resistant Steel)
For heat-resistant stainless steel (austenitic heat-resistant steel) used at high temperatures, the selected welding rods should primarily meet the heat crack resistance of the weld metal and the high-temperature performance of the welded joint.
(V) Key Point Five (Corrosion-Resistant Stainless Steel)
For corrosion-resistant stainless steel operating in various corrosive media, welding rods should be selected according to the medium and operating temperature, ensuring their corrosion resistance (performing corrosion performance tests on the welded joints).
(VI) Key Point Six
For austenitic stainless steel working under low-temperature conditions, the low-temperature impact toughness at the operating temperature of the welded joint should be ensured, thus pure austenitic welding rods are used, like A402, A407.
(VII) Key Point Seven
Nickel-based alloy welding rods can also be selected, such as using a nickel-based welding material with 9% Mo to weld Mo6 type super austenitic stainless steel.
(VIII) Key Point Eight: Selection of Welding Rod Flux Types
The welding of austenitic stainless steel has its unique characteristics, and the selection of welding rods for austenitic stainless steel is particularly important. Through long-term practical experience, it has been proven that using the above measures can achieve different welding methods for different materials and different welding rods for different materials.
The selection of stainless steel welding rods must be based on the base metal and working conditions, including operating temperature and contact media. This has a great guiding significance for us, as it is only through this that we can achieve the expected welding quality.