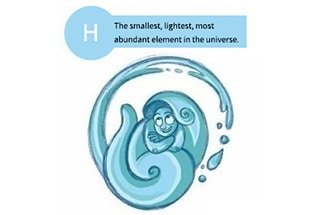
Decarburization in high-speed steel can drastically reduce product quality, causing early failure, quenching cracks, and reduced fatigue strength. But what causes this detrimental effect? Contaminants in salt baths, moisture in the air, and improper heating processes all play a part. By understanding these factors and implementing preventative measures, such as thorough cleaning and precise temperature control, we can significantly enhance the lifespan and performance of steel tools. Dive into this article to explore effective strategies to combat decarburization and protect your high-speed steel investments.
Decarburization of workpieces during salt bath heating and quenching is inevitable for various reasons.
If the decarburization layer is relatively thin, the subsequent grinding process can completely remove it, resulting in no issues.
However, if the decarburization layer cannot be fully removed, the quality of the product will significantly deteriorate.
Decarburization refers to the reduction in carbon content on the surface of steel during heating, or the combustion of carbon with oxygen to form CO2 (C + O2 → CO2).
The process of decarburization occurs when the carbon on the surface of steel reacts with oxygen, hydrogen, etc. at high temperatures, leading to changes in the surface composition and potentially impacting the quality of heat treatment.
It is mainly shown in the following aspects.
The decarburization of a quenched workpiece can reduce its surface hardness and negatively impact its service life.
For instance, a screw groove milling cutter made of W18Cr4V steel with dimensions of φ40mm x 1mm, as shown in Fig. 1, demonstrates the effects of decarburization during heat treatment. The microstructure of the tooth tip surface displays a white crystalline decarburization layer with a microhardness of 338HV, a small amount of black troostite transition zone with a microhardness of 627HV, and a combination of normal tempered martensite and a small amount of carbide with a microhardness of 825HV.
It is noted that the decarburization layer is distributed along the tooth profile. This indicates that decarburization occurred during the heat treatment process. The result is a milling cutter that experiences early wear due to its low hardness.
Fig. 1 quenching and decarburization of screw groove milling cutter
Quenching decarburization results in a difference in the carbon concentration between the inner and outer layers of the workpiece, leading to unequal transformation during the heat treatment process and varying rates of volume expansion and contraction.
This, in turn, creates significant structural and thermal stresses, which increase the likelihood of microcracks forming at stress concentration points in the workpiece.
The decarburization of workpieces significantly affects their fatigue strength, particularly for tools that are subjected to alternating stress in a reciprocating manner. This sensitivity to life results in surface peeling, fracture, and other forms of premature damage as the main characteristics of failure.
The use of quenched molten salt at high temperatures can result in reactions between sulfates, carbonates, and water, causing oxidation and decarburization in mild cases and corrosion pitting in severe cases.
Furthermore, the surface of the workpiece is processed using copper sulfate for chemical copper plating as required by the process.
If the surface copper plating layer is heated to high temperatures prior to removal, it will also result in corrosion of the workpiece surface after quenching.
To maintain the cleanliness of the salt bath and prevent decarburization of quenched parts, many factories add an appropriate deoxidizer to the molten salt. However, it’s important to note that improper construction can lead to the burning of the workpiece.
As seen in Fig. 2, an M2 steel screw slot milling cutter with a diameter of 46mm and a thickness of 1mm was scrapped due to burning caused by the deoxidizer ferrosilicon.
The metallographic structure at the point of burning was identified as secondary ledeburite, with a black belt of troostite (a carbon-poor area) at the junction with the normal structure, as depicted in Fig. 3.
Fig. 2 appearance of burned milling cutter
Fig. 3 metallography of burned milling cutter
The most noticeable effect of decarburization is a decrease in the lifespan of the tool, or in severe cases, rendering it unusable.
The following are the primary causes of decarburization during salt bath quenching:
(1) Harmful impurities, such as Na2SO4, BaSO4, Na2CO3, CaCO3, and BaCO3, present in the salt bath that promote decarbonization.
(2) Increased water solubility in the air leads to easier decarbonization.
(3) Rust on the workpiece or quenching fixture.
(4) The heating process in the salt bath increases the oxide content and oxygen content, which contributes to decarburization of the workpiece.
(5) Nitrate buildup on the quenching hook or the presence of chloride salt mixed with nitrate will also increase the oxygen content in the salt bath.
(6) Inadequate deoxidation of the salt bath or incomplete removal of slag.
(7) Salt bath aging and failure to replace it in a timely manner.
There are several methods to determine if a salt bath decarburizes. The easiest method is using a file, but it requires a wealth of hands-on experience. Other methods include the steel foil test, chemical analysis, hardness test, and microstructure determination. Here is a brief overview of each.
For decarburization through salt bath quenching, a V-shaped groove is filed with a file having a hardness of 66HRC or greater, as the hardness of the part is lower than the core. The depth of the groove determines the extent of decarburization.
Some tool manufacturers determine the hardness and decarburization layer of high-speed steel tools using self-made files. These files are made of 4341 high-speed steel and undergo surface strengthening processes such as QPQ.
After quenching the sample or test piece in a salt bath, it is heated to 700 ℃ and immersed in 20% hydrochloric acid for several minutes. The piece is then cleaned and observed.
The decarburized part and the non-decarburized part have different corrosion resistance, resulting in the decarburized part appearing white. The depth of the decarburized layer can be determined by examining it with a magnifying glass.
Measure the hardness distribution across the cross section of the sample that underwent salt bath heat treatment. If the hardness of the surface differs from the core, the decarburized layer can be determined by identifying where the hardness is equal to the core hardness.
Some individuals believe that a hardness boundary of 823HV0-1 is used to determine if high-speed steel has undergone decarburization after heat treatment. The distance from this boundary to the surface represents the depth of the decarburized layer.
A steel foil (consisting of a 1.0% carbon steel strip with a thickness of 0.5mm, width of 30mm, and length of 150mm) is placed in a salt bath and heated for the specified time under the conditions outlined in Table 1. After heating, it is quenched in 10-30 ℃ flowing tap water for cooling and broken by hand to assess its breaking strength. The decarburization and deterioration of the salt bath are then determined based on the criteria outlined in Table 2.
Table 1 heating time of steel foil in salt bath
Salt bath heating temperature / ℃ | 800 | 900 | 1000 | 1100 | 1200 | 1300 |
Heating time / min | 20 | 15 | 10 | 5 | 3 | 2 |
Table 2 determination criteria for steel foil
Character | Breaking condition | Carbon content of steel foil (%) | Decarburization rate of steel foil (%) | Is the salt bath suitable |
1 | Brittle when broken | >0.60 | 30~40 | ○ |
2 | Elastic when broken | 0.40~0.50 | 50~60 | △ |
3 | Only after twists and turns can it break | 0.20~0.30 | 70~80 | × |
4 | The twists and turns are endless | <0.20 | 80~90 | × |
As illustrated in Fig. 4, the grain size on the surface layer of a high-speed steel workpiece that has undergone quenching and decarburization is relatively coarse.
Fig. 4 metallography of decarburized milling cutter
(1) The rust that has formed on the electrode handle and fixture must be removed prior to quenching.
(2) The slag that has accumulated due to the oxide in the molten salt or any floating objects on the surface of the liquid must be removed promptly.
(3) Regardless of whether the workpieces are dry or not, they must be baked and dried completely.
(4) Salt that is attached to the workpiece or fixture, or scattered salt, should not be brought into the salt bath due to the high risk of oxidation, and must be prevented and removed.
(5) The alkalinity of the molten salt is improved by preventing it from reacting with the atmosphere.
To prevent and minimize the spread of disease, the following steps can be taken:
① The temperature of the salt bath should be kept as low as possible.
② The size of the salt bath surface area should be minimized.
③ The furnace cover should be covered if quenching is not taking place.
④ The surface of the bath liquid should be covered with a circulating nitrogen or inert gas flow.
(6) If the furnace is inactive for an extended period, the salt solution should be drained, dried, broken into small pieces, and stored in a dry location. If a large number of black mud or inclusions are found, they should be discarded and not used any longer.
(7) The slag should be fully deoxidized and removed completely and thoroughly.
(8) A non-deoxygenated, long-acting salt or a 5% MgF2 high-temperature salt bath (95% BaCl2) should be used.
(9) Salt purchased from reputable sources should be sampled and tested before being brought into the factory and stored, and can only be used after it has passed the qualifications.
(10) Effective site management should be practiced and potential decarbonization factors should be dealt with in a timely manner.
Currently, the salt bath furnace remains the primary heating equipment for heat treatment of high-speed steel.
Although the development of vacuum furnaces has been rapid, they cannot fully replace the salt bath furnace.
For an extended period, the advantages of both will complement each other and coexist, but eventually the salt bath will phase out.
It is crucial to pay attention to the quality of salt bath quenching at present, particularly to prevent decarburization.
By closely monitoring each step, it is possible to ensure minimal to no decarburization, thus ensuring the longevity of tools.