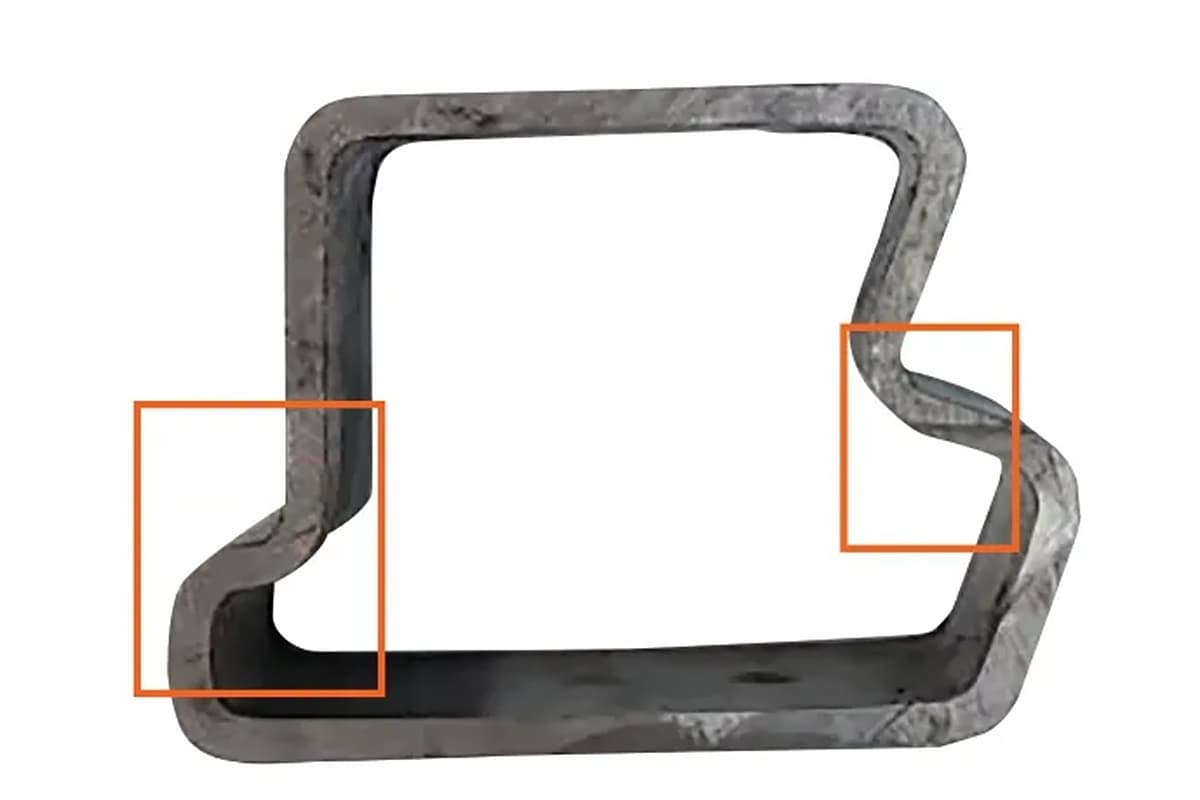
Have you ever wondered how the steel in your car or the pipes in your home are made? This article dives into the fascinating world of forging and rolling, two essential metal processing techniques. Learn the key differences, advantages, and which method suits your needs best.
Forging and rolling are two fundamental metal processing techniques that have been instrumental in shaping the manufacturing landscape. While both methods involve the application of force to reshape metal, they differ significantly in their approach, equipment, and impact on material properties. This article provides an in-depth exploration of these critical metalworking processes, their variations, and their implications for product quality and manufacturing efficiency.
Forging, a process dating back to ancient times, involves the application of localized compressive forces to plastically deform metal into a desired shape. This technique can be further categorized into free forging, where the metal is shaped between flat dies, and closed-die forging, which utilizes specially designed die cavities to produce more complex geometries. Advanced variations such as precision forging and near-net-shape forging have emerged to minimize material waste and subsequent machining operations.
Rolling, on the other hand, is a continuous or semi-continuous process where metal is passed between rotating rolls to reduce its cross-sectional area and achieve desired dimensions and properties. The process can be classified into flat rolling, used for producing sheet and plate products, and profile rolling, which creates various structural shapes. Specialized techniques like ring rolling for seamless rings and thread rolling for fasteners have also been developed to meet specific industry needs.
Both forging and rolling offer distinct advantages in terms of material properties and production capabilities:
Forging:
Rolling:
The choice between forging and rolling depends on various factors, including the desired part geometry, production volume, material properties requirements, and economic considerations. For instance, forging is often preferred for critical components in aerospace and automotive industries where superior mechanical properties are paramount. Rolling, conversely, is the go-to process for producing sheet metal, structural shapes, and rail tracks where consistent cross-sectional properties are crucial.
Recent advancements in both techniques have further expanded their capabilities and efficiency. Computer-aided simulation and design tools now allow for optimized die designs in forging and roll pass sequences in rolling, minimizing trial-and-error approaches. Additionally, the integration of automation and sensors has led to improved process control and quality assurance in both methods.
As the manufacturing industry continues to evolve, understanding the nuances of forging and rolling becomes increasingly important for engineers, designers, and industry professionals. By leveraging the strengths of each technique and considering their impact on material properties, product quality, and production efficiency, manufacturers can make informed decisions to optimize their metalworking processes and maintain a competitive edge in the global market.
A pressure processing method in which a metal blank is passed through the gap between a pair of rotating rolls with various shapes, causing the cross-section of the material to decrease and its length to increase as a result of the compression from the rolls. This method is the most commonly used production technique for steel and is mainly used to produce profiles, plates, and tubes.
According to the movement of rolled parts, rolling can be divided into longitudinal rolling, cross- rolling and skew rolling.
Longitudinal rolling
The longitudinal rolling process is a process in which metal passes between two rolls that rotate in opposite directions and produces plastic deformation between them.
Cross-rolling
The movement direction of the rolled piece after deformation is consistent with the roll axis direction.
Skew rolling
The rolling piece moves in a spiral, the rolling piece and the roll axis are not with a special angle.
The rolling process can improve the quality of steel by destroying the casting structure of the steel ingot, refining the grain of the steel, and eliminating microstructural defects. This leads to a denser steel structure and improved mechanical properties, particularly in the rolling direction.
Additionally, high temperature and pressure during rolling can weld together any bubbles, cracks, or looseness that may have formed during casting.
Forging is a sophisticated metal forming process that employs specialized forging and pressing equipment to apply controlled pressure on metal billets, inducing plastic deformation and producing forgings with precise mechanical properties and geometries. This advanced manufacturing technique not only eliminates inherent casting defects but also optimizes the metal’s microstructure during the forming process.
The preservation of continuous metal flow lines during forging results in superior mechanical properties compared to castings of the same material. This intrinsic advantage makes forgings the preferred choice for critical components subjected to high loads and harsh operating conditions, as well as for simpler geometries that could alternatively be fabricated from rolled plate, extruded profiles, or welded assemblies.
Types of Forging
Forging processes can be categorized into three main types:
Key Features and Advantages
Forging offers several distinct advantages over casting:
(1) Forgings exhibit superior mechanical property consistency in both axial and radial directions compared to rolled products. This enhanced isotropy translates to significantly improved fatigue resistance and overall longevity. The microstructural uniformity in forgings results in more predictable and reliable performance under varying stress conditions.
The figure below illustrates the metallographic distribution of eutectic carbides along different orientations in a Cr12MoV rolled sheet, highlighting the anisotropic nature of rolled products.
(2) Regarding deformation efficacy, forging imparts a substantially higher degree of plastic deformation compared to rolling. This intensified deformation is particularly effective in breaking down and redistributing eutectic carbides, leading to a more refined and homogeneous microstructure. The severe plastic deformation during forging also contributes to grain refinement and the elimination of porosities, further enhancing material properties.
(3) While forging generally incurs higher processing costs than rolling, the investment is often justified for critical components. Forging is indispensable for:
• High-load or impact-resistant parts
• Components with complex geometries
• Applications demanding stringent mechanical properties or dimensional accuracy
The superior mechanical properties and microstructural integrity of forged parts often result in extended service life and improved reliability, potentially offsetting the initial higher cost through reduced maintenance and replacement frequencies.
(4) Forged parts are characterized by continuous and uninterrupted metal flow lines that conform to the part’s geometry. This continuous grain flow significantly enhances the component’s strength, particularly in areas subject to high stress concentrations.
In contrast, machining operations performed on rolled products can disrupt these flow lines, creating potential stress concentration points and reducing the overall fatigue life of the workpiece. This is particularly critical in applications involving cyclic loading or impact stresses.
The image below comparatively illustrates the metal flow lines in cast, machined, and forged workpieces, emphasizing the superior grain structure continuity in forged components.