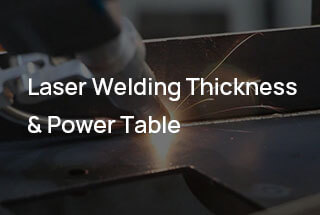
Imagine transforming your welding operations with the power of lasers. The world of laser welding offers a variety of options, including fiber, diode, disc, and CO2 lasers, each with unique advantages and applications. This article explores the key differences between these laser types, helping you make informed decisions for optimal welding performance. From material compatibility to speed and precision, discover how choosing the right laser can revolutionize your manufacturing processes. Dive in to learn which laser suits your needs best and how to achieve superior weld quality.
When choosing a laser welding light source, various factors such as the welding material, joint geometry, speed, and others must be taken into account.
In the manufacturing industry, the correct selection of a laser source is a practical challenge that manufacturers must address due to the widespread use of laser welding.
Currently, the available laser sources in the market include optical fiber, pulsed Nd: YAG, diode, disc, and CO2 laser sources. (Note: The CW Nd: YAG laser source has been largely replaced by optical fiber and disc lasers, and thus is not discussed in this paper).
The choice of a laser source must consider several factors, including the welding material, joint geometry, welding speed, geometric tolerance, system integration requirements, and of course, budget constraints.
Each laser source has unique characteristics that can meet different welding requirements. In some cases, they can also be substituted.
CO2 laser
The CO2 laser, operating at a wavelength of 10.6 μm with power outputs ranging from 1 to 20 kW, is a well-established laser technology. Since the 1980s, it has been the dominant laser source for high-power materials processing applications, particularly in cutting and welding of thick materials.
Fiber laser
Fiber lasers are highly efficient, diode-pumped systems that utilize a small-diameter, silica-based optical fiber doped with rare-earth elements as the active gain medium. The laser source is integrated within the fiber itself, eliminating the need for complex optical alignment and enhancing overall system stability.
With the small core diameter of the optical fiber coupled to the focusing optics, fiber lasers can achieve spot sizes as small as 10 μm, enabling high-precision processing. These compact lasers are available in two primary configurations: low-power (< 300 W) single-mode for fine welding and cutting, and high-power multi-mode for industrial welding and cutting applications.
Diode laser
Recent advancements in diode laser technology have led to their emergence as viable welding sources. Key developments include increased power output of individual diode emitters, improved micro-channel cooling techniques, and progress in micro-optics for efficiently coupling laser output into optical fibers with diameters below 1000 μm. These improvements have significantly enhanced beam quality and power density, making diode lasers increasingly attractive for welding applications, particularly in heat-conduction limited welding scenarios.
Disc laser
The disc laser, also known as the thin-disk laser, utilizes a flat, thin disk of Yb:YAG crystal as the gain medium in a CW laser configuration. This design effectively addresses the thermal management issues inherent in traditional rod lasers. The active medium, typically 0.25 mm (0.01 inches) thick, is cooled by a heat sink on its rear surface, allowing for efficient heat removal.
This innovative architecture enables disc lasers to achieve output powers up to 10 kW while maintaining excellent beam quality (M² < 1.5). The combination of high power and superior beam quality makes disc lasers particularly suitable for deep penetration welding and high-speed cutting applications.
Pulsed Nd:YAG laser
Pulsed Nd:YAG lasers employ a single Nd:YAG crystal rod as the gain medium, excited by flash lamps to generate high peak power pulses with relatively low average power. For example, a system with 35 W average power can produce peak powers of up to 6 kW. The combination of high peak power and narrow pulse widths (typically in the millisecond range) allows for precise control of energy input and heat-affected zone size.
This characteristic makes pulsed Nd:YAG lasers ideal for applications requiring high-quality welds in heat-sensitive materials or thin sections, such as in medical device manufacturing or electronics industry. The ability to manipulate pulse parameters offers flexibility in optimizing weld quality and minimizing thermal distortion.
The choice of laser can be categorized into three groups based on penetration: less than 0.01 inches, between 0.01 to 0.03 inches, and more than 0.03 inches.
Generally, multiple laser sources can be used to complete welding, however, for performance and budget reasons, only one or two light sources may be chosen.
The final decision may also be influenced by various other factors, such as the quality of the sample, geographical considerations, after-sales service, preferences of system integrators, and popularity.
The Pulsed Nd: YAG laser is the most commonly used laser, followed by the fiber laser. In terms of component assembly, joint shape, material, and coating, the entire welding process must be precisely controlled, making the Pulsed Nd: YAG laser the best choice.
With its high peak power, the Pulsed Nd: YAG laser can produce a welding beam with a spot size larger than 1000 microns, providing great flexibility in choosing the spot size and maximizing the process window of welding while ensuring necessary tolerances in the production environment.
The fiber laser is the only continuous-wave laser in this category, and it can produce a spot size after beam focusing of less than 25 microns, providing the high power density required for welding. However, to maintain cost competitiveness in the field of micromachining, the power of fiber lasers is generally limited to 200W, which restricts its maximum spot size and power density.
The size of the soldered joint typically does not exceed 75 microns, which is one of the biggest limitations of fiber lasers. In actual production, it is often difficult to ensure a ± 15mm error range when adjusting joints or components according to fit tolerance and superposition tolerance.
Fiber lasers are mainly used in the lap welding of thin materials with high requirements for welding joints to ensure stability. The fiber laser uses a 150mm focal length lens that can produce light spots with a diameter of less than 25 microns, providing ample processing space. With lap welding, the fiber laser can produce a weld with a penetration depth of 0.01 in or higher at a high speed, with a penetration depth of 0.004in obtainable by a 200W single-mode fiber laser at speeds up to 50 in/s.
On the other hand, the Pulsed Nd: YAG laser can meet almost all applications except for thin foil welding. Its large spot size, pulse width, and peak power range allow for adjustment and optimization to meet various welding requirements.
The application classification of pulsed Nd: YAG laser and fiber laser is still applicable, but the range is limited. The Pulsed Nd: YAG laser is mainly used for spot welding, while fiber lasers with a power of approximately 500W and a spot diameter of 0.01 μm can be used in butt welding and fillet welding with low tolerance. The cost-performance of the Pulsed Nd: YAG laser is relatively high.
Lasers with power levels of 500W and 25W produce different weld penetration at varying welding speeds. The peak power ensures penetration performance, while the average power determines the welding speed of seam welding.
Diode lasers with power ranging between 500W and 800W are suitable for welding components with large tolerances, but the speed is generally slower than that of fiber and disc lasers. However, their large tolerance can make up for this drawback.
All types of lasers are suitable for this range. The penetration depth of the Pulsed Nd: YAG laser is around 0.05 in (1.27 mm), while other laser types can reach up to 0.25 in (6.35 mm) and some even exceed 0.5 in (12.5 mm).
Generally speaking, the parts suitable for Pulsed Nd: YAG laser welding in this range are relatively small, such as pressure sensors with seam welding.
The automotive industry requires a wide range of welding applications, and optical fiber, CO2, disc, and diode lasers can all be used. In terms of speed and penetration, the automotive industry covers almost all welding applications.
The key differences among laser sources are beam quality, brightness, and wavelength.
The beam quality refers to the laser’s focusing ability, and brightness refers to the power density in the focused beam.
For instance, the CO2 laser and fiber laser have similar beam quality, thus if other parameters are equal, they can produce light spots with the same diameter.
However, the wavelength of the fiber laser source is one-tenth that of the CO2 light source, meaning the spot diameter that it can produce is also one-tenth that of the CO2 light source. The fiber laser source also has better beam quality and brightness.
In laser welding, the beam quality and brightness directly impact the penetration depth and speed, but do not have as direct an effect on welding stability and tolerance.
Thus, it is important to find a balance between welding performance and quality, and the width of the process window. It’s worth noting that while beam quality can be reduced to meet specific needs, poor beam quality cannot be improved.
At a 0.25-inch penetration, the welding speeds of the above-mentioned lasers are quite similar. Optical fibers and discs are faster than CO2, while diodes are slower.
Welding with high-power lasers typically requires two shifts, making the cost of purchasing the laser a consideration in the selection process. While CO2 laser is widely used and familiar to many users, its single welding cost is significantly higher than that of fiber, disc, and diode lasers.
Compared to plasma and arc welding, laser welding has more advantages in welding applications requiring penetrations of more than 0.25 inches, as it can greatly reduce thermal deformation. This reduction helps maintain the geometric shape of the part, eliminating the need for reshaping. However, matching parts may cause issues at this thickness. To overcome this, a process flow of wire filling or a combination of laser welding, plasma welding, and arc welding can be used.
There are various types of laser sources available for laser welding, each with its unique features and suitability for specific requirements.
It is crucial for users to comprehend thoroughly which laser source can best fulfill their welding needs.
To obtain a welding system, the optimal approach is to collaborate with the system supplier, who can determine the most appropriate laser for you.
You can also reach out to various laser manufacturers and provide them with welding samples to determine the optimal solution.
When selecting a laser, it’s important to keep in mind that welding must be balanced in terms of penetration, speed, stability, accommodating the production parts and tolerance.