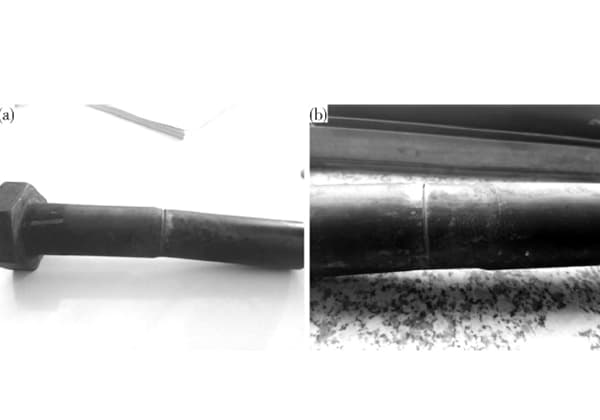
Why did a high-temperature bolt in a turbine fail after thousands of hours in operation? This article dives into the intricate analysis of a fractured bolt used in a power plant’s steam turbine, examining physical and chemical properties to uncover the root cause. Through detailed inspection, the article reveals how material brittleness and coarse grain structure led to the bolt’s failure, offering insights and preventative measures for future occurrences. Learn the critical factors that engineers must consider to ensure the durability of turbine components under extreme conditions.
The domestic thermal generator assembly capacity and thermal efficiency have been constantly improving, leading to a rise in steam pressure in steam turbines. This places higher demands on the high-temperature component materials of the steam turbine.
For instance, high-temperature bolts play a crucial role in ensuring the cylinder split’s airtightness. These bolts are typically made of heat-resistant materials with exceptional high-temperature performance.
One commonly used material for these bolts is 20Cr1Mo1VNbTiB steel, a pearlite heat strength steel that boasts high endurance strength and good anti-relaxation performance.
During the operation of a power plant’s generator unit, a high-temperature bolt in the gate failed and broke. The unit had been in operation for 47341 hours, and the bolt was made of 20Cr1Mo1VNbTiB steel with specifications of M56mm × 4mm × 310mm.
Researchers conducted a thorough inspection and analysis of the broken bolt and compared it to an unbroken bolt from the same batch and material to determine the cause of the failure. They hope to prevent similar incidents from happening in the future.
Fig. 1 Macro appearance of the broken bolt
Fig. 2 Macro morphology of fracture surface of the fractured bolt
The overall and cross-sectional morphology of the broken bolt are depicted in Figures 1 and 2, respectively.
The fracture is observed to be located on the bolt rod, approximately 130mm from the end face. The cross-section appears flat, granular, without any signs of plastic deformation, and exhibits a typical brittle fracture.
An inspection of the bolt’s external surface reveals that the thread surface is smooth and free of any dents, cracks, rust, burrs, or other defects that could cause stress concentration.
A wire cutting sample was taken from the center of both the broken bolt and the comparison bolt. After smoothing the cut surface with sandpaper and cleaning it with alcohol, a full spectrum vertical direct reading spectrometer was used to analyze their chemical composition.
The experimental results showed that the chemical composition of both samples met the requirements for 20Cr1Mo1VNbTiB steel as specified in DL/T 439-2018 Technical Guidelines for High Temperature Fasteners in Fossil Fuel Power Plants.
Both the broken bolt and the unbroken bolt underwent hardness, tensile, and U-notch impact sample testing. The tensile tests were conducted at room temperature using a universal material testing machine, and the results included tensile strength, yield strength, and elongation after fracture. The impact absorption energy was measured at room temperature using an impact testing machine, and the Brinell hardness was measured using a Brinell hardness tester. The test results are presented in Table 1.
Table 1 Mechanical Property Test Results of Bolts
Sample | Yield strength Rp0.2/MPa | Tensile strength Rm/MPa | Elongation after fracture A/% | Impact absorbed energy/J | Hardness/HB | |
End face Transverse load surface 20mm from the end face | ||||||
Measured value of broken bolt | 953 | 1071 | 15 | 25 | 290 | 278 |
Measured value of unbroken bolt | 952 | 1066 | 17 | 86 | 266 | 269 |
standard value | ≥735 | ≥834 | ≥12 | ≥39 | 252~302 |
The test results indicate that the hardness, tensile strength, yield strength, and elongation after fracture of the bolt meet the standard requirements. However, the impact absorption energy of the broken bolt was found to be only 25J, significantly lower than the standard requirements. This suggests that the material of the broken bolt is brittle and has low resistance to impact loads.
In contrast, the impact absorption energy of the unbroken bolt was 86J, which meets the standard requirements.
The end faces of both the fractured and unbroken bolts, as well as the cross-sections of the fracture surfaces of the fractured bolts, were examined for macrostructure according to the recommended method in DL/T 439-2018, as depicted in Figure 3.
Fig. 3 Macro morphology of bolt end face
Examination under different lighting angles reveals that the fracture end face and cross-section of the bolt exhibit patches of polygonal particles with varying colors and brightness. With the naked eye, one can observe that the microstructure is composed of macro coarse grains with an average diameter greater than 2mm, as confirmed by magnifying glass.
The formation of coarse grains in 20Cr1Mo1VNbTiB steel is related to the production process, such as manufacturing and heat treatment, and is a result of overheating. This can occur if the temperature during hot working exceeds Ac1 (the initial temperature for pearlite to austenite transformation) or if the steel is repeatedly heated above the austenitic recrystallization temperature and then rapidly cooled.
In a long-term high-temperature and high-pressure environment, the carbides in the overheated structure gradually precipitate, reducing the material’s impact toughness.
Take samples from the bolt and screw for metallographic inspection, as shown in Fig. 4.
Fig. 4 Microstructure of bolt
The microstructure of the fractured bolt has been determined to be bainite, with a grain size of grade 1 and a cross-distributed row bainite in the crystal that exhibits a frame structure.
The unbroken bolt, on the other hand, has a fine crystalline bainite microstructure with a grain size of grade 5.
The results of the metallographic inspection reveal that the grain size of the broken bolt does not comply with the requirements stated in the DL/T 439-2018 Technical Guidelines for High Temperature Fasteners of Dali Power Plant, which mandate a grain size of grade 5.
The broken bolt’s fracture morphology was examined using a scanning electron microscope.
Fig. 5 Fracture Micromorphology and Energy Spectrum Analysis Results of Broken Bolts
The fracture morphology is depicted in Fig. 5a, and the entire fracture surface is covered by a dense oxide layer. Energy spectrum analysis identified the oxide as Fe2O3, as shown in Fig. 5b.
The fracture of the broken bolt during operation caused the fracture surface to be exposed to high temperatures for an extended period, leading to the formation of a dense oxide film on the surface. This makes it challenging to directly observe the fracture information of the bolt from the fracture surface.
The researchers studied the fracture morphology of the materials through the fracture surfaces of tensile and impact samples to determine the fracture mode of the bolts.
Fig. 6 Fracture Micromorphology of Tensile and Impact Specimens
The fracture surfaces of tensile and impact samples were analyzed using a scanning electron microscope. The fracture morphology of the samples is shown in Fig. 6.
Figures 6a) and 6b) depict the tensile and impact fracture morphologies at low magnification. The shear lip area of the tensile fracture section is minimal, accounting for approximately 15% of the fracture area. The majority of the section is dominated by a radiation area, with very little fiber area present. The macro morphology of the fracture suggests that the material’s toughness is poor.
Figure 6c) illustrates the tensile fracture morphology under high magnification observation. The fracture surface is primarily cleavage, with a small number of dimple inclusions. The overall fracture surface morphology conforms to the characteristics of quasi-cleavage fracture.
Fig. 6d) displays the impact fracture morphology under high magnification observation. The section is predominantly a radiation area, and the entire section is mostly a cleavage surface.
Fig. 6 indicates that the sample’s fracture belongs to cleavage fracture, indicating that the material is highly brittle.
The results of the physical and chemical inspections reveal that the chemical composition, room temperature tensile properties, hardness, and other indicators of the bolts meet the standard requirements.
However, the fracture structure of the bolts has coarse grains with a grade 1 grain size and a frame structure of bainite cross-distributed in the grains, which contributes to the material’s brittleness.
The fracture analysis of the tensile and impact fracture surfaces indicates that the material underwent cleavage fracture, with an impact absorbed energy far lower than the standard requirements. This further confirms the material’s brittleness.
High-temperature bolts in steam turbines operate under challenging conditions, such as high temperatures, high stress, and steam corrosion. As a result, the bolt materials must have high high-temperature creep endurance strength, a low linear expansion coefficient, good anti-relaxation performance, strong stress corrosion resistance, low notch sensitivity, and good oxidation resistance.
However, the fractured bolt material’s coarse grain structure results in excessive brittleness, making it unable to withstand the impact stress caused by start-up, shutdown, and unit load fluctuations.
The high-temperature bolt of the turbine has coarse grains and has been in operation for a prolonged period under high-temperature and high-pressure conditions, leading to the gradual precipitation of carbides and a decrease in material impact toughness.
As a result, the high-temperature bolt experiences brittle fracture under impact stress caused by unit start-up, shutdown, and fluctuation in unit load.
Therefore, it is recommended that thermal power plants conduct 100% ultrasonic inspection and 100% hardness inspection on the high-temperature bolts of the turbine during maintenance, and replace any bolts that do not pass these inspections.