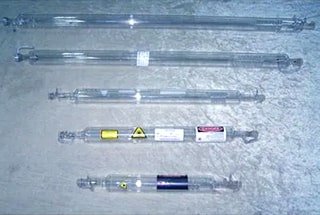
Imagine a future where laser cutting technology revolutionizes the manufacturing industry, boosting efficiency and precision like never before. This article explores how advancements in laser cutting are reshaping sectors from lithium batteries to 3D printing. Readers will learn about the current market trends, technological innovations, and the profound impact of laser processing equipment on various industries. Prepare to discover how these cutting-edge machines are setting the stage for a new era in manufacturing.
In the short term, laser cutting equipment will still be the largest component of the laser processing equipment market, while the continuous expansion of lithium batteries and photovoltaics will bring considerable incremental market for laser processing equipment.
From a macro-processing perspective, the primary cutting equipment in industry includes laser cutters, flame cutters, plasma cutters, waterjet cutters, wire cutters, and shearing machines.
As industrial development progresses, demands for cutting quality, precision, and efficiency continually escalate. Among all cutting methods, laser cutting stands out for its comprehensive advantages.
Historically, the high cost of laser cutting machines has been a significant barrier to their adoption in downstream applications. However, in recent years, the falling prices of domestically-produced lasers have driven down the cost of these cutting machines, making lasers an increasingly popular choice among manufacturers.
Given that the potential for further price reductions in lasers is now relatively small, and the replacement and iterative markets for laser cutting equipment remain vast, general-purpose laser cutting equipment will continue to be the primary market within laser processing equipment in the short term. The market size is expected to maintain a steady growth trend.
Specialized laser processing equipment for lithium batteries utilizes the following laser processing techniques during the manufacturing process:
In the preliminary phase: the anode and cathode tabs are laser cut and shaped, laser cutting of electrodes, laser slitting of electrode sheets, and laser cutting of separators.
During the intermediate phase: laser cleaning of electrodes/tabs, and laser welding of the casing, top cover, sealing pins, and tabs.
In the final phase: laser welding of connecting pieces during battery pack assembly, and laser marking of batteries.
The unique advantages of laser processing, such as non-consumable, no burr or dust, flexible automation integration, high production efficiency, and good process stability, make it irreplaceable in lithium battery production.
Looking at the mid-term, the industrial welding and cleaning markets are vast, yet the penetration of laser welding and cleaning in downstream industries remains low.
They are expected to take the baton from laser cutting equipment and become the primary growth drivers in the laser processing equipment market.
Automated laser welding equipment.
Across the broad spectrum of industrial manufacturing, automated laser welding has been implemented in the production of various products including automobiles, high-speed trains, ships, airplanes, and rockets.
Taking the automobile industry, where laser welding is primarily applied, as an example, laser welding has been used in the manufacture of automobile bodies as early as the 1980s.
In the early 21st century, German brands such as Mercedes, BMW, Audi, and Volkswagen were the first to use laser welding technology in the body manufacturing sector of their domestic joint venture companies.
Today, laser welding production lines have become standard equipment in body welding workshops. Laser welding can bring significant economic benefits to the automobile industry.
Compared to resistance spot welding, which is most frequently used in body assembly, the primary advantages of laser welding include reducing body weight and saving production costs.
Resistance spot welding consumes accessories such as electrode caps, electrode rods, and tools during production, whereas laser welding has virtually no accessory consumption.
In the future, laser welding will open up a broader market in the manufacture of large metal components. In the field of microstructure welding, the contact-free, high-precision, and flexible characteristics of laser welding make it capable of executing precise micro-welding for various 3C products, further expanding the laser welding market.
Handheld laser welding equipment.
Apart from automated laser welding equipment, the advancement in handheld laser welding technology has broadened the application of laser welding.
Automated laser welding equipment, with its large footprint, high equipment cost, and lack of flexibility, has led to the emergence of handheld laser welding equipment as the ideal solution for metal processing manufacturers.
After years of development, handheld laser welding machines have gradually overcome earlier issues such as large size, high precision requirements, and potential safety hazards.
Current air-cooled handheld laser welders are now similar in size to traditional manual arc welders. Swing spot technology significantly reduces the requirement for workpiece fitting gaps, and the inclusion of sensors greatly enhances equipment safety.
Most importantly, the price of handheld laser welding machines has decreased from hundreds of thousands in the early years to tens of thousands today.
This makes handheld laser welding incredibly attractive to industries such as advertising, mold repair, stainless steel bathroom fixtures, sheet metal cabinets, electrical cabinets, stainless steel doors and windows, and wardrobe furniture.
Traditional industrial cleaning methods include mechanical cleaning, chemical cleaning, dry ice cleaning, shot blasting cleaning, ultrasonic cleaning, and more.
Compared with other cleaning methods, laser cleaning offers substantial advantages in terms of damage to the workpiece, cleaning efficiency, consumable costs, and cleaning results.
Most importantly, it doesn’t require any ozone-depleting CFC-based organic solvents, produces no pollution or noise, and is harmless to humans and the environment, making it a “green” cleaning technology.
Laser cleaning equipment is similar to laser welding equipment. Compared to laser cutting and marking, the downstream application scenarios are diverse and complex, requiring more personalized equipment. The promotion of this equipment relies on technological advancements.
The rapid development of the laser processing equipment industry in recent years has also spurred advancements in laser cleaning technology and a reduction in equipment prices. Both large-scale automated laser cleaning equipment and handheld laser cleaning devices have seen numerous successful applications.
It’s foreseeable that laser processing technology will remain a dominant material processing technique for a considerable amount of time in the future.
The scientific and industrial communities’ explorations in laser processing technology have never ceased.
In terms of cutting-edge laser applications, both laser micro-nano machining in the micro-manufacturing sector and laser 3D printing in the macro-manufacturing sector have the potential to further expand the market space for laser processing equipment.
As a non-contact processing method, lasers have unique advantages in micro and nano fabrication.
Lasers have always been crucial in the semiconductor lithography field, for example, the light source used by DUV lithography machines is provided by excimer lasers, while EUV lithography machines require CO2 lasers as the initial light source.
With the increasing demand for precision processing in the microscopic domain by industries such as semiconductors and displays, laser processing technology may continue to provide the best solutions.
Material processing and manufacturing can fundamentally be categorized into three types: subtractive manufacturing, formative manufacturing, and additive manufacturing.
Subtractive manufacturing refers to the use of machines such as lathes, mills, planers, and grinders to cut and shape materials to meet design specifications.
Formative manufacturing involves processes such as casting, forging, and welding to produce products, with the material weight remaining relatively constant.
Additive manufacturing, also known as 3D printing, employs technologies such as photopolymerization, selective laser sintering, and fused deposition to gradually build up material, shaping it into the desired form.
Industrial applications of 3D printing account for 65%-70% of the market, with over half of these applications currently in the aerospace sector. This is partly because some aerospace components have complex structures that are difficult to manufacture using traditional methods.
Moreover, the overall value of aerospace products is high, making them less sensitive to the cost of individual components and more accepting of 3D printing as a manufacturing method.
Currently, 3D printing is particularly suitable for small-scale, customized production in areas like aerospace, fulfilling design requirements for lightweight and functional components.
As 3D printing technology develops and cost control improves, it is expected to find broader application in large-scale industrial production.
Due to the high cost of mass production, additive manufacturing is still used as a supplement to subtractive and formative manufacturing, addressing manufacturing scenarios where traditional methods fall short.
Although additive manufacturing cannot yet match the production efficiency of subtractive and formative manufacturing, given its primary function in creating high-value products, its total value will undoubtedly continue to rise.
As a major part of additive manufacturing, the market potential for laser additive manufacturing equipment is substantial.