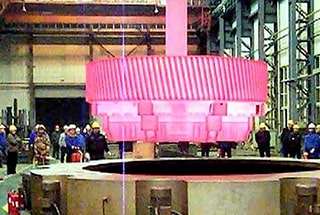
Why do some gears fail despite advanced gas nitriding techniques? This article delves into the critical steps and common pitfalls in the gas nitriding process for gears, from the importance of thorough pre-cleaning to the effects of cooling rates on gear distortion. Readers will gain practical insights on optimizing the gas nitriding process to enhance gear performance and longevity, ensuring high-quality results every time.
Gas nitriding has several advantages over ion nitriding, including ease of operation, high process repeatability, a simple equipment structure, and the ability to achieve automatic control of the process.
One of the most significant benefits of gas nitriding is the improved temperature and atmosphere uniformity during the process.
In particular, the depth of the nitriding layer on the tooth root and pitch circle of small module gears is more uniform during gas nitriding compared to ion nitriding.
In recent years, the gas nitriding process has seen significant advancements, such as the development of pre-oxidation and gas nitriding processes and various other gas nitriding techniques.
These advancements have reduced the production cycle time between ion nitriding and gas nitriding to varying degrees.
However, it’s essential to pay attention to potential issues during the process of controlling the atmosphere during gear nitriding.
Before undergoing gas nitriding and charging, the gear must be thoroughly cleaned to ensure that no water or impurities are present.
If this step is not properly completed, the resulting nitrided surface may have an uneven appearance and dark spots may appear.
Although there is no significant difference in nitrided layer depth, hardness, or metallographic structure between the speckled areas and normal areas, these speckles can significantly impact the overall appearance quality of the gear.
The macro morphology of these irregular speckled areas was analyzed using an ultra-depth of field stereomicroscope (as seen in Figure 1).
Fig. 1 macro morphology of gear end face speckle area
The microscopic morphology of the spotted area was analyzed using a scanning electron microscope (as seen in Figure 2). The areas that appeared white to the naked eye were observed under the scanning electron microscope.
Fig. 2 micro morphology of gear end face speckle area
The chemical composition of the spotted area with abnormal appearance on the gear end face was compared and analyzed with the normal area on the gear end face. The results of this comparison are presented in Table 1.
Table 1 comparison results (mass fraction) (%) of chemical composition determination in micro area of gear end face
Detection location: | C | N | O | Na | Al | Si | P | S | C | K | Ca | Cr | Mn | Fe | |
Zone M | Normal surface | 0.22 | 0.98 | – | – | – | 0.26 | – | – | – | – | – | 0.19 | 0.63 | 97.72 |
0.21 | 0.99 | – | – | – | 0.24 | – | – | – | – | – | 0.25 | 0.57 | 97.73 | ||
Zone A | White spot surface | 0.43 | 1.03 | 2.91 | – | – | 0.22 | – | – | – | 0.09 | 0.21 | 0.19 | 0.35 | 94.59 |
0.38 | 0.89 | 2.58 | – | 0.07 | 0.18 | 0.05 | 0.11 | 0.08 | 0.10 | 0.25 | 0.12 | 0.31 | 94.86 | ||
Peeling flat bottom | 0.28 | 0.73 | 8.59 | – | 0.09 | 0.56 | 0.04 | 0.08 | 0.10 | 0.12 | 0.06 | 0.33 | 1.40 | 89.61 | |
0.39 | 0.84 | 11.59 | 0.15 | 0.10 | 0.67 | 0.13 | 0.11 | 0.09 | 0.12 | 0.06 | 0.34 | 1.71 | 83.71 | ||
Zone B | Dark patches | 1.03 | 0.58 | 29.53 | 0.50 | 0.10 | 0.51 | 0.05 | 0.90 | 0.39 | 1.21 | 0.18 | 0.26 | 0.51 | 64.25 |
0.80 | 0.55 | 28.27 | 0.38 | 0.04 | 0.53 | 0.07 | 1.04 | 0.36 | 1.33 | 0.11 | 0.20 | 0.50 | 65.84 | ||
White round spot | 0.62 | 0.83 | 3.29 | 0.25 | 0.16 | 0.39 | 0.10 | 0.15 | 0.20 | 0.22 | 0.60 | 0.29 | 0.58 | 92.33 | |
0.96 | 0.66 | 5.05 | 030 | 0.16 | 0.60 | 0.06 | 0.14 | 0.23 | 0.30 | 0.96 | 0.32 | 1.09 | 89.16 |
As seen in Table 1, the content of the O element in the abnormal spotted area is higher than that in the normal area. In addition to a higher O content, it also contains trace amounts of Na, S, Cl, K, Ca, Mg, Al, and other elements that come from the residue of water, cleaning agents, and shaving oil.
This analysis shows that the appearance of gas nitriding is caused by poor cleaning before the nitriding process. Hence, the following points should be given special attention during the cleaning of gas nitriding gears:
The distortion that occurs during gear nitriding is influenced by several factors, including gear shape, machining residual stress, gear material, nitriding tooling, nitriding process, and the cooling speed of the gear after nitriding.
In typical production, people tend to focus on the first few factors, but often overlook the cooling speed of the gear after nitriding. This is especially relevant for the inner hole of thin-walled gears, which are particularly sensitive to the cooling speed after nitriding.
For example, consider the gear shown in Figure 3.
Fig. 3 gear size
The inner hole size before nitriding was φ 52-0.02-0.035mm. Table 2 presents the relationship between the gear cooling time and the expansion of the inner hole.
Table 2 Relationship between gear cooling time and inner hole expansion
Cooling time after nitriding / h | Internal hole expansion / mm | Pass rate (%) | Remarks |
≥6 | 0.012~0.021 | 75 | The inner hole size exceeds the upper tolerance |
4~5 | 0.008~0.012 | 99 | The inner hole is basically within the size range |
≤4 | 0.005~0.01 | 78 | Inner hole size out of tolerance |
The test results show that the distortion trend of the inner holes of the gear at different cooling speeds is consistent and generally increases, but the extent of distortion varies. When the cooling rate after nitriding is slow, the inner hole distortion is larger, and when the cooling rate after nitriding is fast, the inner hole distortion is smaller.
To ensure stable distortion of the gear inner hole and improve the qualification rate of the gear inner hole after nitriding, the cooling speed of the gear during gas nitriding must be carefully controlled.
If a gas leak occurs in the gas nitriding furnace due to sealing or other issues, the surface of the workpiece will become oxidized. This oxidation does not affect the internal quality of the workpiece but does not meet the appearance quality standards. In such a situation, the reduction process outlined in Table 3 can be used for repair.
Table 3 repair process of oxidized workpieces
Repair temperature / ℃ | Repair time / min | Ammonia decomposition rate (%) |
480~500 | 30~40 | 30~40 |
The appearance of the gear after gas nitriding, gas leakage oxidation, and reduction is depicted in Figure 4.
Fig. 4 appearance of gears before and after gas ammonia leakage reduction
It’s important to note that during the appearance reduction process, the nitriding temperature must not exceed the first nitriding temperature. Typically, the nitriding temperature during reduction should be about 20°C lower than the last nitriding temperature. The duration of the reduction process can be adjusted based on the extent of oxidation.
The above three problems and solutions highlight the importance of paying attention to every detail in the heat treatment process. With careful consideration of each step, it is possible to effectively resolve any issues that may arise.