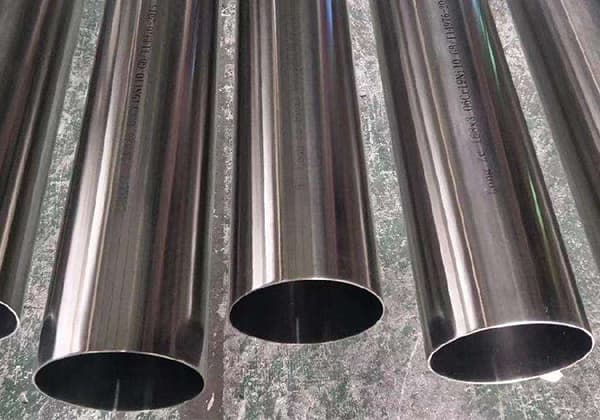
What makes austenitic stainless steel so resilient and versatile? The secret lies in its heat treatment process. This article unpacks the intricate steps and scientific principles behind enhancing its properties. By reading, you’ll uncover how different temperatures and treatments affect the steel’s structure, leading to improved corrosion resistance and strength. Dive in to understand how precise heat management can optimize the performance of this crucial material in various applications.
With the advancement of metallurgical technology, various high-quality stainless steels are continually emerging. Despite the metallurgical industry’s ability to constantly develop superior steel grades, appropriate heat treatment is necessary to optimize the functionality of stainless steel.
During the heating and cooling processes of different steel grades, the transformation of the matrix structure varies, as does the generation and transition of carbides, nitrides, and intermetallic compounds, all of which differently influence the performance of stainless steel.
Therefore, the appropriate heat treatment process should be selected based on the type of steel and intended application during the heat treatment of stainless steel.
Austenitic stainless steel has an austenite matrix structure. During the heating and cooling process, no martensitic phase transformation occurs, hence there is no hardenability.
The purpose of austenitic heat treatment is to enhance corrosion resistance, mitigate the adverse effects brought by the secondary phase, relieve stress, or soften the material that has already undergone work hardening.
(1) Precipitate Generation Temperature
(2) Precipitation and Dissolution of Alloy Carbides
1) Carbon Solubility
For 304 (18Cr-8Ni) steel, the carbon solubility at 1200℃ is 0.34%, at 1000℃ is 0.18%, and at 600℃ is 0.03%.
The carbon content in 304 steel does not exceed 0.08%. Above 1000℃, carbon dissolves in austenite. Given the small radius of carbon atoms, as the temperature decreases, carbon precipitates along the grain boundaries.
2) Intergranular Chromium Depletion
Carbon Solubility: As temperature drops, solubility decreases.
Carbon Atom Radius: Smaller atomic radius means lower solubility, leading to precipitation along the grain boundaries.
Stability: Precipitated carbon atoms are unstable and form stable compounds with chromium and iron, such as Cr23C6 or (FeCr)23C6.
Atomic Diffusion Rate: The smaller radius of carbon atoms results in a higher diffusion rate. Conversely, the larger radius of chromium atoms results in a lower diffusion rate.
(3) Sigma Phase
1) Formation Conditions:
– Prolonged heating in the temperature range of 620~840℃.
– The addition of ferrite-forming elements, such as Titanium (Ti), Neodymium (Nd), etc.
– Using welding rods that have a high content of ferrite-forming elements in the weld seam.
– In austenite with Manganese (Mn), Nitrogen (N) replacing Nickel (Ni).
2) Adverse Effects:
– Reduction in plasticity, especially impact toughness.
– The sigma phase is a rich intermetallic compound, its formation can easily lead to intergranular corrosion, and pitting in Chloride (Cl-) mediums.
(4) Delta Ferrite
1) Formation Conditions:
In cast chromium-nickel austenitic stainless steel, the cast state’s chemical composition is uneven, leading to regions rich in ferrite-forming elements.
In the weld structure of some austenitic stainless steels.
2) Beneficial Effects:
Containing 5-20% delta ferrite can reduce intergranular corrosion.
It enhances the yield strength.
Under low-stress conditions, it can decrease the susceptibility to stress corrosion.
During welding, it reduces the likelihood of thermal cracking.
3) Adverse Effects:
During pressure processing, cracking may easily occur due to the different deformation capabilities of the two structures.
(1) Solution Treatment
1) Solution Treatment Temperature: 950-1150℃
2) Insulation Time: 20-30% longer than general alloy steel.
3) Cooling: Rapid cooling is required in the carbide formation temperature range (450-850℃).
The following principles are applicable for cooling methods:
JIS | Maturation temperature in Celsius. | Cold Working Method |
SUS 403 | 1010-1150 | Rapid Cooling |
SUs 304H | Above 950 | Rapid Cooling |
SUS 304L | 1010-1150 | Rapid Cooling |
SUS 321 | 920-1150 | Rapid Cooling |
SUS 321H | Cold working requires a hardness over 1095. | Rapid Cooling |
Hot working necessitates a hardness exceeding 1050. | Rapid Cooling | |
SUS 316 | 1010-11S0 | Rapid Cooling |
SUS 316H | Above 985 | Rapid Cooling |
SUS 316L | 1010-1150 | Rapid Cooling |
SUS 316JI | 1010-1150 | Rapid Cooling |
SUS 316JIL | 1010-1150 | Rapid Cooling |
SUS 301 | 1010-1150 | Rapid Cooling |
SUS 302 | 1010-1150 | Rapid Cooling |
SUS 309 S | 1030-1180 | Rapid Cooling |
SUS 310 S | 1030~1180 | Rapid Cooling |
SUS 347 | 980~1150 | Rapid Cooling |
SUS 347H | Cold processing of 1095 and above | Rapid Cooling |
High-temperature processing of 10S0 and above. | Rapid Cooling | |
SUS 303 | 1010-1150 | Rapid Cooling |
SUS 305 | 1010-1150 | Rapid Cooling |
SUS 30SM | 1010-1150 | Rapid Cooling |
SUS 317 | 1010-1150 | Rapid Cooling |
SUS 317L | 1010-1150 | Rapid Cooling |
SUH 31 | 950-1150 | Rapid Cooling |
SUH 309 | 1030-1150 | Rapid Cooling |
SUH 310 | 1030-1180 | Rapid Cooling |
SUH 330 | 1030-1180 | Rapid Cooling |
(2) Stabilizing Treatment
The stabilizing treatment is a heat treating method used for austenitic stainless steel containing Nd or Ti.
1) Stabilizing treatment temperature: Higher than the dissolution temperature of chromium carbides (450-870℃) but lower or slightly higher than the dissolution temperatures of TiC and NbC (750-1120 ℃). The general recommendation is 870-950 ℃.
2) Soaking time: 2-4 hours (depending on the shape of the workpiece, alloy elements, etc.). The soaking time for those with a thickness or diameter of 25mm is 2 hours, and an additional hour is added for larger sizes.
3) Cooling: Slow cooling rates, such as air cooling or furnace cooling.
(3) Stress Relief Annealing
1) The stress relief annealing process for austenitic stainless steel should be selected based on the material’s properties, the operating environment, the purpose of stress elimination, and the size and shape of the workpiece.
2) The purposes of stress relief annealing are:
3) Stress Corrosion Cracking
Steel Grade | Heat treatment | Residual stress in kgf/mm2 | The time at which rupture occurs in boiling 42% MgCl2 (at 154 degrees Celsius). | ||||
Circumferential direction | Longitudinal direction | ||||||
304 | Cooling state(Tensile strength 115.9 kg/mm2) | 32.4 | 48.3 | 7.5 | Fracture | ||
Half-hard condition (Tensile strength 93.2 g/mm2) | – | – | 6 | Fracture | |||
540℃ | 24hr | Air Cooling | – | – | 7.5 | Fracture | |
650 | 0.5 | Air Cooling | – | – | 22 | Fracture | |
650 | 8 | Air Cooling | – | – | 14.5 | Fracture | |
745 | 0.5 | Air Cooling | 1.3 | 5.9 | 245 | Minor fracture | |
745 | 0.5 | False Cooling | – | – | 292 | A rupture | |
870 | 0.5 | Air Cooling | – | – | >292 | No fracture | |
870 | 0.5 | False Cooling | – | – | >292 | No fracture | |
870 | 24 | Air Cooling | – | – | >292 | No fracture | |
316 | 1/4H Cooling Condition(Tensile Strength 80.4 kg/mm2) | 36.7 | 14.7 | 7.5 | Fracture | ||
On-site heat treatment and cooling correction(Tensile strength 64.3 kg/mm2) | 11.9 | – | 7.5 | Fracture | |||
540℃ | 24h | – | 31.5 | – | 7.5 | Fracture | |
650 | 0.5 | – | 27.3 | – | 7.5 | Fracture | |
650 | 8 | – | – | – | 14.5 | Fracture | |
745 | 0.5 | – | 18.7 | – | 22 | Fracture | |
745 | 0.5 | – | 16.3 | – | 22 | Fracture | |
745 | 8 | – | – | – | 22 | Fracture | |
790 | 0.5 | – | 7.3 | – | 24 | Fracture | |
840 | 0.5 | – | 2.5 | – | >240 | No fracture | |
870 | 0.5 | Air Cooling | 2.5 | 5.8 | >292 | No fracture | |
870 | 0.5 | False Cooling | – | – | >292 | No fracture | |
870 | 24 | Air Cooling | – | – | >292 | No fracture |
4) Stress Relief Annealing Method
Types of Materials Method Usage conditions and the purpose of stress relief. | Type I (Ultra-Low Carbon) 00Cr19Ni10 00Cr17Ni14Mo2 | Class II (Including Stable Elements) 0Cr18Ni10Ti 0Cr18Ni11Nb | Type III (Other) 0Cri8Ni10 0Cr17Ni12Mo2 |
For high-stress corrosion environments. | A·B | B·A | ① |
For medium-stress corrosion environments. | A·B·C | B·A·C | C① |
For low-stress corrosion environments. | A·B·C·D·E | B·A·C·D·E | C·E |
Mitigate localized stress concentration. | E | E | E |
Applicable in intergranular corrosion environments. | A·C② | A·C·B② | C |
Eliminate substantial residual stress post-processing. | A·C | A.C | C |
Relieve stress incurred during the machining process. | A·B·C | B·A·C | C③ |
In situations involving significant residual stress from machining and stress generated during use, as well as large-section, extensive welded components. | A·C·B | A·C·B | C |
Ensure dimensional stability of components. | F | F | F |
Note: The methods in the table are listed in order of priority.
Hold time: For every 25mm, hold for 1-4 hours. Longer hold times are needed at lower temperatures.
Notes: