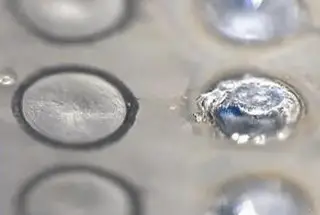
Choosing the right laser welding method can dramatically impact your manufacturing efficiency and product quality. Have you ever wondered whether continuous or pulse laser welding is better for your needs? This article breaks down the differences, comparing aspects like power, efficiency, and application suitability. By the end, you’ll understand which technique aligns best with your specific welding requirements, ensuring optimal performance and cost-effectiveness. Dive in to make an informed decision that could streamline your operations and enhance your output quality.
Continuous welding of the galvanized sheet by the semiconductor laser
Pulsed welding of stainless steel plate with Nd 3 +: YAG laser
Lasers can be classified through various methods, with wavelength and active medium being two primary categorization approaches. Wavelength-based classification divides lasers into infrared, visible, and ultraviolet categories, while active medium classification includes CO2 lasers, fiber lasers, Nd3+:YAG solid-state lasers, Nd3+:YAG disc lasers (proprietary to Trumpf), direct semiconductor lasers, and dye lasers, among others. Additionally, lasers are distinguished by their mode of operation: continuous wave (CW) or pulsed.
The fundamental operation of a laser involves beam output through oscillation within a resonant cavity. However, high-frequency oscillation can produce multiple outputs. The transition from pulsed to continuous wave operation occurs when the output frequency reaches a critical threshold.
While there is no universally accepted standard for delineating between continuous wave and pulsed lasers in academia or industry, a general consensus exists:
In metal cutting applications, typical frequency parameters vary by laser type. For example, IPG and Raycus fiber lasers commonly operate at 5000 Hz, while earlier Nd3+:YAG solid-state laser cutting machines typically function at 300 Hz. CW lasers are generally characterized by their power output, whereas pulsed lasers are defined by single pulse power, average power, pulse width, and frequency.
The relationship between these parameters for pulsed lasers can be expressed as:
Average Power = Single Pulse Power × Pulse Width × Frequency
This equation allows for cost-effective performance evaluation and optimization of pulsed laser systems in industrial applications.
In metal laser welding, the choice of laser source significantly impacts the welding process and results. Traditionally, Nd3+:YAG solid-state lasers have been the go-to for pulse welding, while fiber lasers have dominated continuous welding applications. However, recent advancements in laser technology are blurring these distinctions, with industrial-grade direct semiconductor lasers gaining traction in continuous welding operations.
Pulse laser welding, typically employing Nd3+:YAG lasers, is characterized by low-frequency, high-energy pulses. For example, a 500W pulse laser can generate single pulse powers exceeding 12kW, resulting in superior penetration depth compared to fiber lasers of equivalent average power. This high peak power allows for effective welding of reflective materials and enables precise control over heat input, making it ideal for applications requiring minimal heat-affected zones (HAZ).
Conversely, continuous wave (CW) lasers, predominantly fiber lasers, deliver high-frequency, stable output with lower single pulse energies. These lasers excel in high-speed welding applications and offer excellent beam quality, allowing for focused energy delivery and consistent weld quality over extended periods. The continuous nature of the beam also facilitates smooth welding of thicker materials and enables advanced techniques such as remote welding.
To illustrate the difference between pulse and continuous welding, consider this analogy: pulse welding resembles pile driving, where each impact is forceful but infrequent, resulting in deep, localized energy transfer. Continuous welding, in contrast, is akin to rapid hammering with an electric nail gun, delivering a steady stream of lower-energy impacts at high speed, ensuring consistent energy distribution along the weld seam.
The selection between pulse and continuous lasers depends on factors such as material properties, joint configuration, production speed requirements, and desired weld characteristics. Modern laser systems often offer hybrid capabilities, combining the advantages of both pulse and continuous modes to optimize welding processes for specific applications.
As laser technology continues to evolve, emerging trends include the development of ultra-short pulse lasers for micro-welding applications and the integration of real-time process monitoring and adaptive control systems to enhance weld quality and consistency in automated production environments.
The beam characteristics of lasers play a crucial role in their cutting performance and applications. Different laser types exhibit distinct beam profiles, which significantly influence their interaction with materials.
Continuous wave (CW) fiber lasers typically produce a Gaussian beam profile. This profile is characterized by a high power density at the beam’s center, which decreases exponentially towards the edges, following a bell-shaped curve. The Gaussian distribution results in a TEM00 (Transverse Electromagnetic Mode) output, offering excellent focusability and high power density at the focal point. This characteristic makes CW fiber lasers particularly effective for high-precision cutting and welding of metals.
In contrast, pulsed lasers generally exhibit a flat-top (or top-hat) beam profile. This profile features a more uniform energy distribution across the beam’s cross-section, with relatively sharp edges. The even power distribution of flat-top beams provides advantages in certain applications, such as surface treatment, heat treating, and some types of welding, where a more uniform energy deposition is desirable.
It’s noteworthy that direct diode lasers (DDLs) also typically produce a beam profile similar to the flat-top distribution. While not the focus of this discussion, DDLs are gaining importance in industrial applications due to their high efficiency and compact design. Their beam characteristics can offer advantages in applications requiring uniform heating or processing over larger areas.
Energy distribution of Gaussian beams
Energy distribution of flat-topped beam
The frequency of light output in continuous welding is extremely high.
When proper welding protection and parameters are used, a uniform and smooth weld can be achieved that does not require grinding or polishing.
Pulse welding, on the other hand, has a lower light frequency and produces a clear, intermittent knocking sound during the welding process. The resulting weld has a flat fish scale appearance, similar to argon arc welding, or full single spot welding spots as needed.
Continuous welding only requires a few parameters to be selected, such as the appropriate welding track, running speed, and power, making it relatively simple. Pulse welding, however, requires a comprehensive consideration of multiple parameters, including pulse width, light output frequency, single pulse power, running speed, and pulse waveform, making it a more complex process.
Pulse welding spot (welding spot)
Continuous welding seam
Furthermore, the fiber laser beam has a high energy density at its optical center. With current technology, the laser beam can be efficiently transmitted through a fiber with a very small core diameter. This makes medium to high-power light lasers ideal for deep penetration welding, producing welds with a high depth-to-width ratio.
The flat-top distribution of pulsed laser beams offers significant benefits for heat conduction welding, particularly in thin-plate splicing welding.
The continuous fiber laser has significantly disrupted the welding industry, capturing substantial market share from CO2 and Nd3+:YAG solid-state lasers. This shift is attributed to its superior characteristics: enhanced stability, reduced power consumption, higher efficiency, exceptional beam quality, and increased energy density. Industry trends indicate this technology will continue to dominate in the foreseeable future.
Despite the continuous fiber laser’s ascendancy, Nd3+:YAG solid-state lasers retain relevance in specialized applications. Their extensive development history and established market presence position them well for niche sectors requiring specific wavelengths or pulse characteristics.
Currently, continuous laser welding excels in deep penetration applications. The automotive parts industry, for instance, extensively utilizes optical fiber lasers for precision welding of components such as transmission gears and engine parts. As fiber laser and direct semiconductor laser technologies advance, we anticipate broader adoption across diverse industries, including aerospace, medical device manufacturing, and advanced electronics.
The high efficiency and stability of continuous laser welding align seamlessly with the manufacturing industry’s push towards Industry 4.0 principles. These lasers integrate well with automated production lines, robotic systems, and real-time process monitoring, enabling smart manufacturing processes that enhance productivity and quality control.
While the market share for pulse welding has decreased, it maintains relevance for specific applications. Fiber lasers have developed quasi-continuous modes that compete with traditional pulse welding, offering high peak powers with controllable heat input. However, pulse welding remains indispensable for heat-sensitive materials, micro-welding applications, and scenarios requiring precise control over the heat-affected zone (HAZ).
The Nd3+:YAG solid-state laser, with its advantages of lower initial cost, user-friendly operation, and simplified maintenance, continues to be a viable option for small to medium-scale operations and job shops. These factors, combined with its established presence in certain industries, ensure the ongoing relevance of pulse welding technology.
As welding technology evolves, we expect to see further innovations in beam shaping, multi-beam solutions, and hybrid welding techniques that combine the strengths of different laser types to address complex manufacturing challenges.
Selecting the optimal welding method between pulsed and continuous laser welding requires a comprehensive evaluation of multiple factors, including initial investment, operational costs, process-specific requirements, production efficiency, and material considerations.
Pulsed laser welding, while exhibiting lower overall energy efficiency, offers precise control over heat input through high single pulse energy. This characteristic makes it particularly suitable for heat-sensitive materials, thin sections, and applications requiring minimal heat-affected zones. However, the specialized equipment and potentially slower production rates can lead to higher operational costs.
Continuous laser welding, in contrast, offers superior energy efficiency and higher production speeds, resulting in lower per-unit costs for high-volume manufacturing. The consistent heat input allows for deeper penetration and is ideal for thicker materials. While the initial investment in continuous laser systems may be higher, the long-term operational costs are generally lower due to improved energy utilization and faster processing times.
The choice between these methods ultimately depends on specific application requirements:
Emerging hybrid systems combining both methods are now offering increased flexibility, allowing manufacturers to optimize their processes for a wider range of applications. As laser technology continues to advance, improvements in beam quality, power efficiency, and control systems are further blurring the lines between these two approaches, potentially leading to more versatile welding solutions in the future.