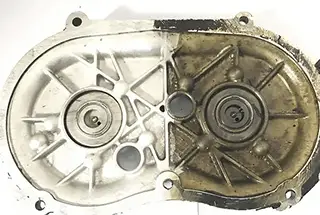
Have you ever wondered why lasers are so precise and powerful? Unlike regular light, lasers have unique characteristics such as high directionality, monochromaticity, coherence, and intensity. This article explores these properties and their applications, from medical instruments to industrial cutting. Dive in to discover how these fascinating beams work and their impact on technology and science.
A typical light source emits light that radiates in all directions and diminishes with increasing distance. This attenuation is mainly because the light from such sources is the result of a multitude of molecules or atoms within the source emitting photons independently during spontaneous radiation. In contrast, lasers amplify incident photons through stimulated emission.
Due to the different mechanisms behind laser generation compared to ordinary light sources, lasers exhibit unique characteristics not shared with conventional light, which can generally be summarized in four aspects: directionality, monochromaticity, coherence, and high intensity.
Lasers emit light through stimulated radiation; each photon maintains the same frequency, phase, and polarization state as the incident light, all under the control of an optical resonator. This control allows the laser beam to propagate strictly along the axis of the resonator with a very small divergence angle, approaching that of parallel light.
The high directionality of lasers is determined by the mechanism of stimulated emission and the restrictive influence of the optical resonator on the direction of the oscillating light beam. Precise data has shown that a laser beam emitted from Earth to the Moon, a distance covering approximately 380,000 kilometers, results in a beam spot on the Moon that is less than 1000 meters in diameter.
This excellent directionality has led to the widespread use of lasers in ranging, communication, and positioning. The high directionality of lasers allows for effective transmission over long distances and focusing into very high power densities, both of which are crucial for laser processing.
The color of light is determined by its wavelength. The width between the two wavelengths at which the intensity is half of the maximum is typically defined as the spectral line’s width. The narrower the spectral line width, the better the monochromaticity of the light. Visible light consists of seven colors, each with a spectral line width of 40 to 50 nanometers.
The monochromaticity of lasers far exceeds that of ordinary light sources. For example, the spectral line width of the red laser light emitted by a helium-neon laser is only 10-8 nanometers, which is significantly more monochromatic than a krypton lamp. Some special lasers have even higher monochromaticity.
The extremely high monochromaticity of lasers virtually eliminates the chromatic dispersion (the variation of refractive index with wavelength) of focusing lenses, enabling the light beam to be precisely focused on the focal point, achieving a high power density. The excellent monochromaticity of lasers provides an advantageous tool for precision instrument measurements and for stimulating certain chemical reactions in scientific experiments.
Coherence principally describes the phase relationships between different parts of a light wave, encompassing two aspects: temporal coherence and spatial coherence. For lasers, the spatial distribution of the light field is typically decomposed into a distribution along the propagation direction (cavity axis) E(z) and a distribution on the transverse cross-section perpendicular to the propagation direction E(x, y).
Thus, laser cavity modes can be divided into longitudinal and transverse modes, representing the longitudinal and transverse light field distributions of the cavity modes, respectively.
The temporal coherence of a laser refers to the phase relationships among points along the direction of beam propagation. In practical applications, coherence time is often used to describe a laser’s temporal coherence. The narrower the spectral line width, that is, the higher the monochromaticity, the longer the coherence time.
Single-mode stabilized frequency gas lasers have the best monochromaticity, typically reaching 106 to 1013 Hz; solid-state lasers have poorer monochromaticity, mainly because their gain curve is wide, making it difficult to ensure single longitudinal mode operation; semiconductor lasers have the worst monochromaticity.
Single-mode operation (mode selection technology) and frequency stabilization are crucial for enhancing coherence. A frequency-stabilized single transverse mode laser emits light that is close to an ideal monochromatic plane wave, i.e., fully coherent.
The spatial coherence of a laser is the phase relationship between points on a plane perpendicular to the direction of beam propagation. It refers to the scale over which light emitted by the beam can converge at a point in space to form interference patterns, and spatial coherence is related to the size of the light source.
An ideal plane wave is fully spatially coherent and has a divergence angle of zero. However, in practice, due to diffraction effects, the smallest beam emission angle achievable by a laser cannot be less than the diffraction limit angle when passing through the output aperture.
To improve a laser’s spatial coherence, it is essential first to restrict the laser to operate in a single transverse mode; second, to select the type of optical cavity appropriately and to increase the cavity length to enhance the beam’s directivity. Additionally, inhomogeneities in the active medium, errors in cavity machining and adjustment, and other factors can also degrade the beam’s directivity.
Due to the excellent directivity of laser beams, the energy emitted is confined within a very narrow solid angle, and the energy is concentrated within a narrow spectral line width. This significantly increases the spectral brightness of lasers compared to conventional light sources. In pulsed lasers, where the energy emission is further compressed into a very short time interval, the spectral brightness can be further enhanced.
Currently, increasing output power and efficiency is an important direction in laser development. Gas lasers, such as CO2, can produce the highest continuous power, while solid-state lasers can produce the highest pulse power.
Especially with the use of optical cavity modulation techniques and laser amplifiers, laser oscillation time can be compressed to very small values (on the order of 10-9 seconds), and the output energy can be amplified, resulting in extremely high pulse power. With mode-locking and pulse width compression techniques, laser pulse widths can be further compressed to 10-15 seconds.
Most importantly, laser power (energy) can be concentrated in a single (or few) modes, thus achieving a very high degree of photon degeneracy. When a laser beam is focused through a lens, it can generate temperatures of several thousand, even tens of thousands of degrees Celsius near the focal point, enabling the processing of all materials.
For instance, high-power CO2 laser cutting machines commonly used in industry adopt focal lengths of 127 to 190 mm, with focal spot diameters ranging from 0.1 to 0.4 mm, and their energy density can reach 10 W/cm2.