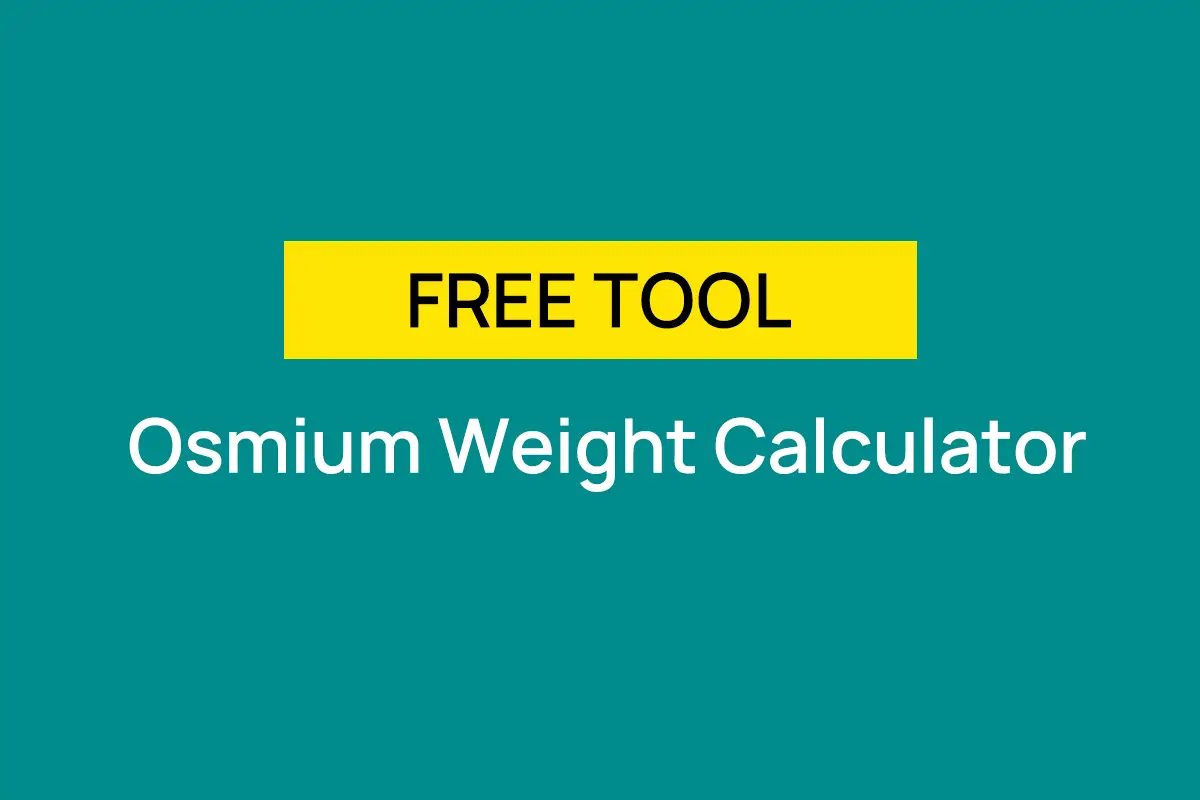
Have you ever wondered why stainless steel is so widely used in engineering and manufacturing? This article explores the eight critical mechanical properties that make stainless steel indispensable: yield strength, tensile strength, yield ratio, elongation, strain hardening index, austenite equilibrium coefficient, martensite transformation point, and grain size. By understanding these properties, you’ll gain insights into the versatility and reliability of stainless steel in various applications. Dive in to discover how these properties affect performance and suitability for your projects.
(mechanical symbol σ0.2, abbreviation YS)
σ0.2=P0.2/F0
A low yield strength of a material means that it is prone to yielding, has minimal bounce-back after being formed, and has favorable properties for die fitting and maintaining shape during forming.
(mechanical symbol σb, abbreviation TS)
σb=Pb/F0
A high tensile strength of a material means that it is resistant to breaking during deformation, making it suitable for undergoing plastic deformation.
(σ0.2/σb)
The yield strength ratio has a significant impact on the formability of materials during stamping.
When the yield strength ratio is low, the stage of plastic deformation from yielding to fracture in sheet metal is prolonged, reducing the risk of fracture during forming, making it easier to stamp.
In general, a low yield strength ratio enhances the resistance to cracking in sheet metal during various forming processes.
Table: Yield ratio of common stainless steel materials
Type of Steel | Yield strength (N/mm2) | Tensile strength (N/mm2) | Yield ratio |
SUS304 | 300 | 670 | 0.45 |
SUS304(Cu) | 295 | 640 | 0.46 |
SU5316 | 312 | 625 | 0.50 |
SUS316L | 245 | 525 | 0.47 |
SUS430 | 350 | 510 | 0.69 |
SUS409L | 241 | 410 | 0.59 |
(mechanical symbol, English abbreviation EL)
Elongation refers to the ratio of the total increase in length of the material from plastic deformation to fracture, compared to its original length. It is expressed as:
A high elongation of a material means that it can undergo greater plastic deformation and has good crack resistance, making it favorable for drawing, flanging, and bulging.
Typically, the flanging coefficient and bulging property (Ericsson value) of a material are directly proportional to its elongation.
The strain hardening index, also known as the “n value,” reflects the cold work hardening of materials and its impact on the formability during stamping.
A high strain hardening index indicates that the material has strong local strain capacity and can effectively prevent local thinning. This means that increasing the instability limit strain results in a more uniform deformation distribution, and the overall forming limit of the material is high during forming.
A(BAL) = 30(C+N)+0.5Mn+Ni-1.3Cr+11.8
The stability of austenite is indicated by the “A value.” A smaller A value means that the austenite is less stable.
The structure of steel is susceptible to changes from cold and hot working, which can affect its mechanical properties.
Ni, Mn, C, and N are common elements that help form and stabilize austenite in stainless steel, especially Ni. An increase in the content of these elements can increase the austenitic equilibrium coefficient and make the austenitic structure more stable.
Cr, Mo, Si, Ti, and Nb are elements that help form and stabilize the ferrite structure. An increase in the content of Cr can reduce the austenitic equilibrium coefficient.
SUS304 stainless steel is a pure austenitic structure with its own stability. After cold working, it becomes hard due to a portion of the austenitic structure changing to martensite, known as cold working induced martensite.
Austenitic stainless steel has a small balance coefficient, making it prone to martensite transformation or further martensite formation during cold working, resulting in a high degree of cold work hardening.
Md(30/50)= 551-462(C+N)-9.2Si-8.1Mn-13.7Cr-29(Ni+Cu)-18.5Mo
The martensite transformation point (Md(30/50)) is the temperature at which 50% of the material undergoes martensite transformation after undergoing 30% true strain from cold deformation. The higher the content of alloy elements in austenitic stainless steel, the lower the martensite transformation point.
Austenitic stainless steel with a lower martensite transformation point is less prone to induced martensite during cold working and has a low degree of cold work hardening.
Cold work hardening in stainless steel is caused by two factors: work hardening due to an increase in dislocations and work hardening due to structural transformation (from austenite to martensite).
SUS430 steel does not undergo structural transformation during deformation and its cold work hardening is solely caused by an increase in dislocations.
In contrast, the cold work hardening of SUS304 steel is mainly due to the transformation from austenite to martensite, with a smaller contribution from an increase in dislocations. This is why the cold work hardening of austenitic stainless steel is more pronounced than that of ferritic stainless steel.
The Ni content has a significant effect on the martensite transformation point in austenitic stainless steel. An increase in Ni content leads to a lower martensite transformation point and a smaller degree of cold work hardening.
The physical meaning of grain size can be understood according to the following formula:
ξ=2N+3
A higher grain size N level means that there are more grains per unit cross-sectional area, making the grain size finer. This results in higher strength and better elongation of the material.
Steel with N>5 (256 grains/mm) is considered fine-grain steel.
Large grain size can increase the plastic strain ratio (R) of the material, but also decreases the yield strength ratio and elongation.
However, with large grains, there can be different orientations on the surface of the sheet metal, leading to unequal deformation and causing the “orange peel” effect on the material surface.
Refining the grain size can reduce the occurrence of orange peel, but if the grain size is too fine, the plastic strain ratio will decrease, and the yield strength ratio and elongation will increase, making it less favorable for forming.