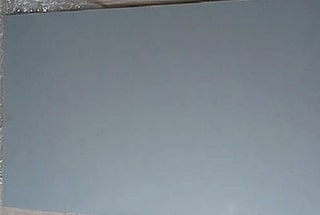
Ever wondered why some metals are perfect for your project while others fall short? In this article, we explore the key factors in selecting the right metal material for your needs. Get ready to uncover practical tips and insights that will help you make informed decisions and achieve optimal results in your mechanical engineering projects.
When selecting materials and manufacturing processes, it is imperative to conduct a comprehensive evaluation based on three critical perspectives: suitability, feasibility, and cost-effectiveness. This multi-faceted approach ensures optimal performance, manufacturability, and economic viability of the final product.
Suitability assessment involves analyzing whether the material’s properties and performance characteristics align with the intended working conditions and functional requirements. This includes evaluating mechanical properties (such as strength, ductility, and fatigue resistance), thermal behavior, corrosion resistance, and any specific attributes crucial for the application.
Feasibility analysis focuses on determining if the chosen manufacturing process is compatible with the selected material. This encompasses considerations such as formability, machinability, weldability, and any limitations or challenges associated with processing the material using available equipment and techniques. Advanced simulation tools and pilot runs may be employed to validate process feasibility and identify potential issues before full-scale production.
Cost-effectiveness evaluation examines the economic aspects of both material selection and manufacturing processes. This involves analyzing raw material costs, processing expenses, energy consumption, tooling requirements, and potential yield rates. Additionally, lifecycle costs, including maintenance, recyclability, and disposal, should be factored into the overall cost-effectiveness assessment.
The principle of suitability is fundamental in material selection, demanding that chosen materials must withstand operational conditions and meet performance requirements. This critical step in material selection ensures optimal functionality and longevity of components.
Material suitability is determined by a comprehensive set of internal quality specifications, including:
When selecting materials, engineers must consider three key factors:
1. Load conditions: This encompasses both the magnitude and nature of applied stresses (static, dynamic, cyclic) and their distribution within the component. Factors such as fatigue, creep, and impact resistance may be critical depending on the application.
2. Service environment: This includes:
3. Performance requirements:
A holistic evaluation of these factors is essential to ensure that the selected materials not only meet but exceed the performance requirements. This approach minimizes the risk of premature failure, optimizes component lifespan, and ensures cost-effective material utilization.
Additionally, emerging considerations in material selection include sustainability, recyclability, and environmental impact, which are becoming increasingly important in modern engineering practices.
Once materials have been selected, the processing technology can generally be determined. However, it’s important to keep in mind that the processing process can alter the properties of materials. Additionally, factors such as the shape, structure, batch size, and production conditions of the parts also play a significant role in determining the material processing technology.
The principle of feasibility requires considering the processability of the materials when selecting them, and materials with good processability should be preferred to minimize the manufacturing difficulty and cost. Each manufacturing process has its own unique characteristics, advantages, and disadvantages.
When parts made of the same material are manufactured using different processes, the difficulty and cost can vary, as can the required material processing performance. For example, forging may not be feasible for parts with complex shapes and large sizes. In such cases, casting or welding may be used, but the material must have good casting or welding performance and the structure must meet the requirements for casting or welding.
In another example, when manufacturing keys and pins through cold drawing, the elongation of the materials and the impact of deformation strengthening on their mechanical properties should be considered.
In addition to meeting the requirements of material use and processing, it’s crucial to consider the cost-effectiveness of materials in metal fabrication and manufacturing processes.
The principle of cost-effectiveness involves selecting materials that offer an optimal balance between performance and price. Performance encompasses the material’s functional properties, typically represented by its service life, mechanical strength, corrosion resistance, and safety factors. The price of materials is determined by both initial and lifecycle costs, including production expenses and long-term operational costs.
Material costs are influenced by multiple factors:
To optimize cost-effectiveness, manufacturers should consider:
The steps for selecting materials and manufacturing processes are as follows:
To evaluate the service conditions of parts, the specific load, stress state, temperature, corrosion, and wear conditions that the parts will experience during use must be determined.
For parts used in normal temperature conditions, the main requirement is for the materials to have adequate mechanical properties. However, for parts used under different conditions, the materials must have specific physical and chemical properties.
If the parts will be used at high temperatures, the materials must have high temperature strength and resistance to oxidation. Parts used in chemical equipment must have high resistance to corrosion. Some instrument parts require materials with electromagnetic properties. For welding structures used in extremely cold areas, requirements for low temperature toughness must be considered.
When used in humid areas, requirements for atmospheric corrosion resistance must be included. The following are the general steps for material selection:
Please note that these are just general guidelines for material selection and the process can be time-consuming and complex.
For important parts and new materials, a significant number of basic tests and trial production processes are required to ensure material safety during selection. For less important and small batch parts, materials are typically selected based on the use experience of similar materials under the same working conditions, and the brand and specification of materials are determined, followed by arranging the forming process.
If the parts are damaged normally, the original materials and forming process can be used. If the damage is due to abnormal early damage, the cause of failure must be determined and appropriate measures taken. If it is a result of the material or its production process, new materials or a new molding process may be considered.
(1) Load conditions
Engineering materials are exposed to various forces during operation, such as tensile stress, compressive stress, shear stress, cutting stress, torque, and impact force, among others.
The mechanical properties and modes of failure of materials are closely tied to the load conditions they are subjected to.
In engineering, it is crucial for machinery and structures to function safely and reliably while meeting their movement requirements.
For instance, the spindle of a machine tool must be able to operate normally without breaking or excessive deformation under stress. Another example is that when a jack lifts a load, the screw must remain straight and balanced without bending suddenly.
The safe and reliable operation of engineering components depends on their meeting the requirements of strength, stiffness, and stability.
There are specific conditions for each of these aspects of materials in material mechanics that must be considered when analyzing stress conditions or selecting materials.
When selecting materials based on stress conditions, it is important to consider not only the mechanical properties of the materials, but also the relevant knowledge of material mechanics to make a scientifically informed choice.
Table 1 Stress, Failure Forms and Required Mechanical Properties of Several Common Parts
Spare parts | Working conditions | Common failure forms | Main mechanical property requirements | ||
Stress category | Load properties | Other forms | |||
Ordinary fastening bolt | Tensile stress and shear stress | Static load | Excessive deformation and fracture | Yield strength Shear strength | |
Transmission shaft | Bending stress Torsional stress | Cyclic shock | Friction and vibration at journal | Fatigue failure, excessive deformation and wear at journal | Comprehensive mechanical properties |
Transmission gear | Compressive stress and bending stress | Cyclic shock | Strong friction, vibration | Wear, pitting peeling, tooth breakage | Surface: hardness, bending fatigue strength, contact fatigue resistance; Center: yield strength, toughness |
Spring | Torsional stress Bending stress | Cyclic shock | Vibration | Loss of elasticity, fatigue fracture | Elastic limit, yield ratio, fatigue strength |
Oil pump plunger pair | Compressive stress | Cyclic shock | Friction, oil corrosion | abrasion | Hardness and compressive strength |
Cold working die | Complex stress | Cyclic shock | Strong friction | Wear and brittle fracture | Hardness, sufficient strength and toughness |
Die-casting die | Complex stress | Cyclic shock | High temperature, friction, liquid metal corrosion | Thermal fatigue, brittle fracture, wear | High temperature strength, thermal fatigue resistance, toughness and red hardness |
Rolling bearing | Compressive stress | Cyclic shock | Strong friction | Fatigue fracture, wear, pitting peeling | Contact fatigue resistance, hardness and wear resistance |
Crankshaft | Bending stress Torsional stress | Cyclic shock | Journal friction | Brittle fracture, fatigue fracture, erosion and wear | Fatigue strength, hardness, impact fatigue resistance and comprehensive mechanical properties |
Connecting rod | Tensile stress and compressive stress | Cyclic shock | Brittle fracture | Compressive fatigue strength, impact fatigue resistance |
(2) Service temperature of materials
Most materials are typically used at room temperature, however, there are also materials that are used at high or low temperatures.
Because of these varying service temperatures, the required properties of the materials also vary greatly.
As the temperature decreases, the toughness and plasticity of steel materials decrease continuously. At a certain point, there is a significant decrease in toughness and plasticity, referred to as the ductile-brittle transition temperature.
When used below the ductile-brittle transition temperature, materials are susceptible to brittle fracture under low stress, which can result in harm. Thus, when selecting steel for low temperature use, materials with a ductile-brittle transition temperature lower than the working conditions should be chosen.
The alloying of various low temperature steels is aimed at reducing carbon content and improving their low temperature toughness.
As temperature increases, the properties of steel materials undergo several changes, including a decrease in strength and hardness, an increase and then decrease in plasticity and toughness, and oxidation or corrosion at high temperatures.
These changes impact the material’s performance and may render it unusable. For example, the service temperature for carbon steel and cast iron should not exceed 480 ℃, while the service temperature for alloy steel should not exceed 1150 ℃.
(3) Corrosion
In the industry, the corrosion rate is commonly used to express the corrosion resistance of materials.
The corrosion rate is measured as the loss of metal material per unit area in a specific amount of time, or as the depth of corrosion in the metal material over time.
Industry commonly uses a corrosion resistance rating system of 6 categories and 10 grades, ranging from Class I with complete corrosion resistance to Class VI with no corrosion resistance, as shown in Table 2.
Table 2 Classification and Rating Criteria for Corrosion Resistance of Metallic Materials
Corrosion resistance classification | Corrosion resistance classification | Corrosion rate, mm/d | |
I | Complete corrosion resistance | 1 | <0.001 |
Ⅱ | Very corrosion resistant | 23 | 0.001~0.005 0.005~0.01 |
III | Corrosion resistance | 45 | 0.01~0.05 0.05~0.1 |
IV | Corrosion resistance | 67 | 0.1~0.5 0.5~1.0 |
V | Poor corrosion resistance | 89 | 1.0~5.0 5.0~10.0 |
VI | Non corrosion resistance | 10 | >10.0 |
Most engineering materials operate in atmospheric environments and suffer from atmospheric corrosion, which is a common issue.
The atmosphere’s humidity, temperature, sunlight, rainwater, and content of corrosive gases greatly impact the corrosion of these materials.
In common alloys, carbon steel has a corrosion rate of 10^-605 m/d in industrial atmospheres, but it can be used after being painted or treated with other protective layers.
Low alloy steel containing elements such as copper, phosphorus, nickel, and chromium has greatly improved atmospheric corrosion resistance and can be used without being painted.
Materials such as aluminum, copper, lead, and zinc have good atmospheric corrosion resistance.
(4) Wear resistance
The following are the factors affecting the wear resistance of materials:
① Material properties: including hardness, toughness, ability to undergo work hardening, thermal conductivity, chemical stability, surface state, etc.
② Friction conditions: including the characteristics of the abrasive material in the friction, the pressure, temperature, speed of friction, the properties of lubricants, and the presence of corrosive conditions.
In general, materials with high hardness are less susceptible to penetration or abrasion by grinding objects, and have a high fatigue limit, resulting in high wear resistance. Additionally, high toughness ensures that even if the material is penetrated or abraded, it will not break apart, further improving its wear resistance.
Therefore, hardness is the primary aspect of wear resistance. It is important to note that the hardness of materials can change during use. For instance, metals that undergo work hardening become harder during friction, while metals that can be softened by heat may soften during friction.
Generally, once the material of a product is determined, the type of forming process is typically identified.
For instance, if the product is made of cast iron, casting should be used; if it’s made of sheet metal, stamping should be the choice; if it’s made of ABS plastic, injection molding is the way to go; and if it’s ceramic parts, the appropriate ceramic forming process should be selected.
However, it’s important to keep in mind that the forming process can also affect the material’s performance, so the final performance requirements of the material must be taken into account when selecting the forming process.
Performance of Product Materials
① Mechanical Properties of Materials
For example, steel gear parts can be cast when their mechanical properties are not critical, but when high mechanical properties are required, pressure processing should be used.
② Service Performance of Materials
For example, when manufacturing flywheel parts for cars and automobile engines, steel die forging should be used instead of open die forging. This is because the high speed of cars and the requirement for smooth driving means that exposed fibers in flywheel forgings could lead to corrosion and affect performance. Closed die forging is preferred over open die forging as it eliminates the flash and prevents cutting off and exposing the fiber structure of the forgings.
③ Technological Properties of Materials
Technological properties include casting properties, forging properties, welding properties, heat treatment properties, and cutting properties. For instance, nonferrous metal materials with poor weldability should be connected using argon arc welding instead of manual arc welding. PTFE, being a thermoplastic material with poor fluidity, is not suitable for injection molding and should only be molded through pressing and sintering.
④ Special Properties of Materials
Special properties include wear resistance, corrosion resistance, heat resistance, conductivity, or insulation. For example, the impeller and shell of an acid-resistant pump should be made of stainless steel and cast. If plastic is used, injection molding is an option. If both heat resistance and corrosion resistance are required, ceramics should be used and molded through the grouting process.
(2) Production Batch of Parts
For mass production of products, the forming process with high precision and productivity should be selected to ensure accuracy and efficiency. Although the equipment required for these molding processes may have a relatively high manufacturing cost, this investment can be offset by the reduction in material consumption per product.
For mass production of forgings, the recommended forming processes include die forging, cold rolling, cold drawing, and cold extrusion.
For mass production of non-ferrous alloy castings, metal mold casting, die casting, and low pressure casting are the recommended molding processes.
For mass production of MC nylon parts, the injection molding process is the preferred choice.
For small batch production, forming processes with lower precision and productivity, such as manual molding, free forging, manual welding, and processes that involve cutting, may be selected.
(3) Shape Complexity and Accuracy Requirements of Parts
For metal parts with complex shapes, particularly those with intricate inner cavities, the casting process is often selected, such as for box, pump body, cylinder block, valve body, shell, and bed components.
Engineering plastic parts with complex shapes are typically produced using the injection molding process.
Ceramic parts with complex shapes can be produced using either the injection molding or casting process.
For metal parts with simple shapes, pressure processing or welding forming processes can be used.
Engineering plastic parts with simple shapes can be produced using blow molding, extrusion molding, or molding processes.
Ceramic parts with simple shapes are usually molded.
If the product is a casting and dimensional accuracy is not a high requirement, ordinary sand casting can be used. For high dimensional accuracy, investment casting, evaporative pattern casting, pressure casting, or low pressure casting can be selected based on the casting material and batch size.
For low dimensional accuracy requirements in forging, free forging is commonly used. For high precision requirements, die forging or extrusion forming is selected.
If the product is plastic and requires low precision, hollow blow molding is preferred. For high precision requirements, injection molding is selected.
(4) Existing Production Conditions
The existing production conditions refer to the current equipment capacity, technical expertise of personnel, and the possibility of outsourcing products.
For instance, when producing heavy machinery products, if there is no large-capacity steelmaking furnace or heavy-duty lifting and transportation equipment on site, the combined process of casting and welding is often used. This involves dividing the large parts into smaller pieces for casting and then welding them together to form larger pieces.
As another example, the oil pan parts for a lathe are typically produced through stamping thin steel plates with a press. If the conditions on site are not suitable for this process, alternative methods should be used.
For instance, if there are no thin plates or large presses on site, the casting process may need to be used. If thin plates are available but there is no large press, an economical and feasible spinning forming process may be used as a substitute for stamping forming.
(5) Consideration of New Processes, Technologies, and Materials
With the growing demands of the industrial market, users have increasingly high requirements for product variety and quality upgrades, leading to a shift from mass production to multi-variety and small batch production. This expands the scope of application for new processes, technologies, and materials.
In order to shorten the production cycle and upgrade product types and quality, it is necessary to consider the use of new processes, technologies, and materials such as precision casting, precision forging, precision blanking, cold extrusion, liquid die forging, superplastic forming, injection molding, powder metallurgy, ceramics, and other static pressure forming, composite material forming, and rapid forming. This will allow for nearly net-shaped parts and significant improvement in product quality and economic benefits.
In addition, to make a reasonable selection of the molding process, it is important to have a clear understanding of the characteristics and application scope of various molding processes, as well as the impact of the molding process on material properties.
The characteristics of various blank forming processes of metal materials are shown in Table 3.
Table 3 Characteristics of various blank forming processes
Casting | Forging | Stamping parts | Weldment | Rolled stock | |
Molding characteristics | Forming under liquid state | Solid plastic deformation | Solid plastic deformation | Connection under crystallization or solid state | Solid plastic deformation |
Requirements for material process performance | Good liquidity and low shrinkage | Good plasticity, small deformation resistance | Good plasticity, small deformation resistance | High strength, good plasticity, good chemical stability in liquid state | Good plasticity, small deformation resistance |
Common materials | Steel materials, copper alloys, aluminum alloys | Medium carbon steel, alloy structural steel | Mild steel, nonferrous metal sheet | Low carbon steel, low alloy steel, stainless steel, aluminum alloy | Low and medium carbon steel, alloy steel, aluminum alloy, steel alloy |
Metal structure characteristics | Coarse grain and loose tissue | The grains are fine, dense and directionally arranged | Forming new streamline organization along the stretching direction | The weld zone is of casting structure, and the fusion zone and overheat zone are coarse | The grains are fine, dense and directionally arranged |
Metal structure characteristics | Coarse grain and loose tissue | The grains are fine, dense and directionally arranged | Forming new streamline organization along the stretching direction | The weld zone is of casting structure, and the grains in the fusion zone and superheat zone are coarse | The grains are fine, dense and directionally arranged |
Mechanical property | Slightly lower than forgings | Better than castings of the same composition | The strength and hardness of the deformed part are high, and the structural rigidity is good | The mechanical properties of the joint can reach or approach the base metal | Better than castings of the same composition |
Structural characteristics | Unrestricted shape, can produce parts with rather complex structure | Simple shape | Light structure and slightly complex shape | Size and structure are generally unrestricted | Simple shape, less changes in horizontal dimensions |
Material utilization rate | high | low | higher | higher | Lower |
Production cycle | long | Short free forging, long die forging | long | Shorter | short |
Production costs | Lower | higher | The larger the batch, the lower the cost | higher | Lower |
Main scope of application | Various structural and mechanical parts | Transmission parts, tools, molds and other parts | Various parts formed by sheet | Various metal structural parts, partially used for parts blanks | Structural blanks |
Application examples | Frame, bed, base, workbench, guide rail, gearbox, pump body, crankshaft, bearing seat, etc | Machine tool spindle, transmission shaft, crankshaft, connecting rod, bolt, spring, die, etc | Automobile body, engine meter housing, electrical instrument housing, water tank, oil tank | Boiler, pressure vessel, chemical vessel pipeline, plant structure, bridge, vehicle body, hull, etc | Smooth shaft, lead screw, bolt, nut, pin, etc |