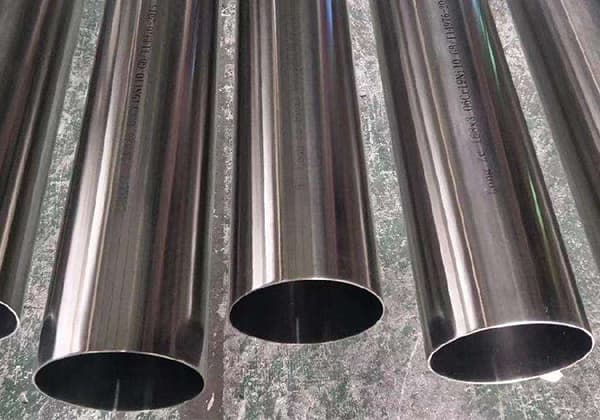
Imagine materials that can revolutionize entire industries—making things stronger, lighter, and smarter. This article explores 50 innovative materials that are set to transform technology and manufacturing. From graphene’s unmatched strength and conductivity to biodegradable plastics reducing pollution, these materials are at the forefront of scientific advancement. By diving into their unique properties and potential applications, you’ll discover how they might reshape the future of everything from aerospace to everyday products. Prepare to be inspired by the limitless possibilities these materials offer.
New materials, also known as advanced materials, refer to those recently developed or under development that exhibit superior performance compared to traditional materials.
They encompass materials that are newly developed or in the process of research, demonstrating exceptional capabilities that outperform conventional materials.
The technology of new materials is crafted in accordance with human intent, through a series of research processes including physical research, material design, processing, and experimental evaluation, all aiming to create innovative materials that meet a variety of needs.
This includes functional positioning, directional positioning, technical positioning, and market positioning:
(1) Composite New Materials
The use of composite new materials dates back to ancient times. Historical examples include straw-reinforced clay and the century-old steel-reinforced concrete, both composed of two different materials. In the 1940s, due to the needs of the aviation industry, glass fiber-reinforced plastics (commonly known as fiberglass) were developed, marking the advent of composite materials. Since the 1950s, high-strength and high-modulus fibers like carbon, graphite, and boron fibers have been developed. The 1970s saw the emergence of aramid and silicon carbide fibers.
These high-strength, high-modulus fibers can be combined with non-metallic matrices like synthetic resins, carbon, graphite, ceramics, rubber, or metallic matrices like aluminum, magnesium, titanium to form unique composite materials. Ultra-high molecular weight polyethylene fibers, known for their exceptional strength and resistance to chemical agents and aging, also excel in high-frequency sonar transmission and seawater corrosion resistance.
These fibers are used in high-frequency sonar fairings for naval vessels, enhancing mine detection and sweeping capabilities. Beyond military applications, they have broad prospects in automobile manufacturing, shipbuilding, medical devices, and sports equipment. Their introduction has garnered significant attention and importance in developed countries.
(2) Superconducting Materials
Some materials exhibit zero electrical resistance at a certain critical temperature, a phenomenon known as superconductivity. Another characteristic of superconductors is their diamagnetism – the inability of magnetic field lines to penetrate a superconductor when it becomes resistance-free. For example, the electrical resistance of common metals like copper decreases with temperature and reaches a certain value near 0K.
In 1919, Dutch scientist Heike Kamerlingh Onnes discovered that mercury’s resistance completely disappears at 4.2K (-269°C), demonstrating superconductivity and diamagnetism. The critical temperature (TC) at which a superconductor’s resistance becomes zero is a key feature. Superconducting material research focuses on overcoming the “temperature barrier” to find high-temperature superconductors.
Practical superconductors like NbTi and Nb3Sn have been commercialized, finding applications in nuclear magnetic resonance imaging (NMRI), superconducting magnets, and large accelerator magnets. SQUIDs, as examples of superconductors in weak electrical applications, play a crucial role in detecting weak electromagnetic signals, unmatched in sensitivity by any non-superconducting device.
However, the low critical temperatures of conventional superconductors, requiring complex and expensive liquid helium (4.2K) systems, have significantly limited their applications. The advent of high-temperature oxide superconductors broke through this temperature barrier, raising the applicable temperature from liquid helium (4.2K) to liquid nitrogen (77K) levels. Liquid nitrogen is a more economical coolant with a higher heat capacity than liquid helium, greatly facilitating engineering applications.
High-temperature superconductors also have considerable magnetic capabilities, capable of generating magnetic fields over 20T. Applications of superconducting materials include power generation, transmission, and storage. Superconducting generators with coil magnets can increase magnetic field strength to 50,000-60,000 Gauss with almost no energy loss, improving single-unit capacity by 5-10 times and efficiency by 50% compared to conventional generators.
Superconducting transmission lines and transformers can transmit electricity to users with minimal loss. For instance, about 15% of electrical energy is lost in copper or aluminum transmission lines in China, amounting to over 100 billion kWh yearly. Switching to superconducting transmission could save enough electricity to replace the need for dozens of large power plants.
Superconducting maglev trains operate using the diamagnetic property of superconductors, which repel magnetic field lines, allowing the superconductor to levitate above a permanent magnet or magnetic field. This maglev effect is used in high-speed superconducting maglev trains, like the one at Shanghai Pudong International Airport. In superconducting computers, the near-zero resistance of superconducting materials allows for densely packed circuits on integrated chips without overheating, significantly boosting computing speed.
(3) Energy Materials
Energy materials include solar cell materials, hydrogen storage materials, and solid oxide fuel cell materials. Solar cell materials, a new energy material, have seen advancements like IBM’s multi-layer composite solar cells with conversion efficiencies up to 40%. Hydrogen, a pollution-free and efficient energy source, faces key challenges in storage and transport. About 50% of the U.S. Department of Energy’s hydrogen research funding is allocated to hydrogen storage technology.
Hydrogen can corrode materials, causing embrittlement and leakage, and poses an explosion risk during transport. Hydrogen storage materials can form hydrides with hydrogen, releasing hydrogen upon heating and recharging after depletion. Current hydrogen storage materials are mainly metal compounds, such as LaNi5H and Ti1.2Mn1.6H3. Research on solid oxide fuel cells is active, focusing on materials like solid electrolyte membranes, cell cathode materials, and organic proton exchange membranes for proton exchange membrane fuel cells.
(4) Smart Materials
Smart materials represent the fourth generation of materials following natural, synthetic polymer, and artificially designed materials. They are a significant direction in modern high-tech new material development. Internationally, numerous technical breakthroughs have been achieved in smart materials. For example, the UK’s BAE Systems developed wire sensors to test strain and temperature on aircraft skins.
The UK also developed a rapid-response shape memory alloy with a lifespan of a million cycles and high output power, useful in brakes with response times as short as 10 minutes. Shape memory alloys have been successfully applied in satellite antennas, medical fields, and more. Other smart materials include piezoelectric materials, magnetostrictive materials, conductive polymers, electrorheological fluids, and magnetorheological fluids, serving as drive components in various applications.
(5) Magnetic Materials
Magnetic materials are categorized into soft and hard (permanent) magnetic materials.
(1) Soft Magnetic Materials
Soft magnetic materials are easily magnetized and demagnetized, losing their magnetism when the magnetic field is removed. Characterized by high magnetic permeability (μ=B/H), they are easily magnetized to high strength in magnetic fields but retain little residual magnetism when the field is removed.
These materials are widely used in electronic technology, particularly in high-frequency applications like magnetic cores, heads, and memory cores, and in electrical engineering for transformers and relay switches. Common soft magnetic materials include iron-silicon alloys, iron-nickel alloys, and amorphous metals. Fe-(3%-4%)Si alloy, the most commonly used soft magnetic material, is used in low-frequency transformers, motors, and generators.
Iron-nickel alloys, such as Permalloy (79%Ni-21%Fe), offer higher magnetic permeability and lower loss than iron-silicon alloys and are used in telecommunications, computers, and control systems. Amorphous metals, differing from typical metals with their non-crystalline structure, are composed of Fe, Co, Ni, and metalloids like B, Si.
Produced by rapidly cooling molten metal to achieve a non-crystalline atomic structure, amorphous metals exhibit excellent magnetic properties and are used in energy-efficient transformers, magnetic sensors, recording heads, and more. Some amorphous metals also have excellent corrosion resistance, high strength, and good toughness.
(2) Permanent Magnetic Materials (Hard Magnetic Materials)
Permanent magnetic materials retain their magnetism after magnetization, even when the external magnetic field is removed. They are characterized by high residual magnetism and high coercivity, making them suitable for permanent magnets used in compasses, instruments, micro-motors, electric motors, recorders, telephones, medical applications, and more. Permanent magnetic materials include ferrites and metal permanent magnets.
Ferrites, widely used for their large volume, broad application, and low cost, have moderate magnetic properties and are suitable for general permanent magnet applications. Metal permanent magnets started with high carbon steel but evolved to higher performance materials like Al-Ni-Co and Fe-Cr-Co alloys; rare earth magnets, such as earlier rare earth-cobalt (Re-Co) alloys (mainly SmCo5 and Sm2Co17 made using powder metallurgy) and widely used niobium-iron-boron (Nb-Fe-B) rare earth magnets. Nb-Fe-B magnets not only offer superior performance but also lack the scarce element cobalt, quickly becoming the representative of high-performance permanent magnets, used in high-performance speakers, electronic water meters, nuclear magnetic resonance instruments, micro-motors, car starter motors, and more.
(6) Nanomaterials
Nanotechnology is an integrated system that combines cutting-edge high technology and science, fundamentally involving understanding and modifying nature at the nanoscale by directly manipulating and arranging atoms and molecules to create new materials. Nanotechnology encompasses seven areas: nanosystem physics, nanochemistry, nanomaterials science, nanobiology, nanoelectronics, nanofabrication, and nanomechanics.
Nanomaterials, named in the 1980s, are solid materials composed of nanoparticles not exceeding 100 nanometers in size. The preparation and synthesis of nanomaterials remain the main research focus, and although some progress has been made in sample synthesis, the large-scale production of bulk samples is still a challenge, making the study of nanomaterial preparation critical for their application.
The materials industry is the basic industry of the national economy, and new materials are the precursors of the development of the materials industry.
Graphene, carbon nanotubes, amorphous alloys, foamed metals, ionic liquids … 20 new materials bring unlimited opportunities for the development of the materials industry.
Today, the scientific and technological revolution is developing rapidly, new material products are changing with each passing day, and the pace of industrial upgrading and material replacement is accelerating.
New materials technology is integrated with nanotechnology, biotechnology, and information technology.
Structural and functional integration and functional materials are becoming more intelligent.
The materials’ low-carbon, green, and recyclable environmentally friendly characteristics have attracted much attention.
Based on the research progress of domestic and foreign well-known research institutions and companies, scientific and technological media reviews, and industry hot spot research, this article has selected 20 new materials.
The following is the detailed information of the relevant materials (in no particular order).
Breakthrough:
Extraordinary electrical conductivity, extremely low resistivity, extremely low and extremely fast electron migration speed, tens of times strength and excellent light transmission over the steel.
Development Trend:
The 2010 Nobel Prize in Physics has made graphene hot in technology and capital markets in recent years.
In the next 5 years, the use of graphene will grow explosively in the fields of photoelectric displays, semiconductors, touch screens, electronic devices, energy storage batteries, displays, sensors, semiconductors, aerospace, military, composite materials, and biomedicine.
Main research institutes (companies):
Graphene Technologies, Angstron Materials, Graphene Square, Forsman Technology, etc.
Breakthrough:
High porosity, low density, light weight, low thermal conductivity, excellent thermal insulation properties.
Development Trend:
New materials with great potential.
They have great potential in the fields of energy conservation and environmental protection, heat insulation in electrical appliances, and construction.
Main research institutes (companies):
Fosman Technology, W.R. Grace, Fuji-Silysia, Japan, etc.
Breakthrough:
High electrical conductivity, high thermal conductivity, high elastic modulus, high tensile strength, etc.
Development Trend:
Electrodes for functional devices, catalyst carriers, sensors, etc.
Main research institutes (companies):
Unidym, Inc., Toray Industries, Inc., Bayer Materials Science AG, Mitsubishi Rayon Co., Ltd. , Forsman Technology, Suzhou First Element, etc.
Breakthrough:
With linear and non-linear optical properties, alkali metal fullerene superconductivity, etc.
Development Trend:
The future has important prospects in the fields of life sciences, medicine, astrophysics, etc., and is expected to be used in photoelectric devices such as optical converters, signal conversions and data storage.
Main research institutes (companies):
Michigan State University, Xiamen Funa New Materials, etc.
Breakthrough:
High strength and toughness, excellent magnetic permeability and low magnetic loss as well as excellent liquid flow.
Development Trend:
Can be used in high-frequency low-loss transformers, structural parts of mobile terminal equipment, etc.
Main research institutes (companies):
Liquidmetal Technologies, Inc., Institute of Metal Research, Chinese Academy of Sciences, BYD, etc.
Breakthrough:
Light weight, low density, high porosity and large specific surface area.
Development Trend:
It has conductivity and can replace application fields where inorganic non-metallic materials cannot conduct electricity.
It has great potential in the field of sound insulation and noise reduction.
Main research institutes (companies):
Alcan (Aluminum Association,USA), Rio Tinto, Symat, Norsk Hydro, etc.
Breakthrough:
With high thermal stability, wide liquid temperature range, adjustable acid and alkali, polarity, coordination ability and etc.
Development Trend:
It has broad application prospects in the field of green chemical industry, as well as biology and catalysis.
Main research institutes (companies):
Solvent Innovation, BASF, Lanzhou Institute of Physics, Chinese Academy of Sciences, Tongji University, etc.
Breakthrough:
It has good biocompatibility, water holding capacity, and wide range of pH stability.
It also has a nano-network structure and high mechanical properties.
Development Trend:
It has great prospects in biomedicine, enhancer, paper industry, purification, conductive and inorganic compound food as well as industrial magnetic compound.
Main research institutes (companies):
Cellu Force (Canada), US Forest Service, Innventia (Sweden), etc.
Breakthrough:
Namometer perocakite has giant magneto-resistance, high ionic conductivity, and plays a catalytic role in the precipitation and reduction of oxygen.
Development Trend:
It will has huge potential in the fields of catalysis, storage, sensors, and light absorption in the future.
Main research institutes (companies):
Epry, AlfaAesar, etc.
Breakthrough:
Changing the traditional industrial processing methods can quickly achieve the formation of complex structures.
Development Trend:
The revolutionary molding method has great prospects in the field of complex structure molding and rapid processing molding.
Main research institutes (companies):
Object, 3DSystems, Stratasys, Huashu Hi-Tech, etc.
Breakthrough:
It changes the rigid and fragile characteristics of traditional glass and realizes the revolutionary innovation of glass flexibility.
Development Trend:
The prospect will be huge in the field of future flexible displays and foldable devices.
Main research institutes (companies):
Corning, Germany SCHOTT, etc.
Breakthrough:
The self-assembly of material molecules will realize the “intelligence” of the material itself.
Changing the previous method of material preparation to realize the material to form a certain shape and structure spontaneously.
Development Trend:
Changing traditional materials preparation and materials repair methods has great prospects in the fields of molecular devices, surface engineering and nanotechnology.
Main research institutes (companies):
Harvard University, etc.
Breakthrough:
Plastics can be naturally degraded and the raw materials come from renewable resources which changing the traditional plastics’ dependence on fossil resources such as oil, natural gas and coal, and also reducing environmental pollution.
Development Trend:
It will replace traditional plastics in the future and has great prospects.
Main research institutes (companies):
Natureworks, Basf, Kaneka, etc.
Breakthrough:
These kind of materials have high strength, low density, and excellent corrosion resistance.
And also have unlimited prospects in aviation and civil fields.
Development Trend:
In the future, the materials will have a wide range of potential applications in light weight, high strength, and corrosion resistance.
Main research institutes (companies):
Harbin Institute of Technology.
Breakthrough:
It has physical properties that conventional materials do not have, such as negative permeability and negative permittivity.
Development Trend:
It has changed the traditional concept of processing according to the nature of the material.
In the future, the characteristics of materials can be designed according to needs, and the potential will be infinite and revolutionary.
Main research institutes (companies):
Boeing, Kymeta, Shenzhen Guangqi Research Institute, etc.
Breakthrough:
In the superconducting state, the material has zero resistance, no current loss, and the material exhibits anti-magnetic properties in a magnetic field.
Development Trend:
If the high-temperature superconducting technology has been broken through in the future, it is expected to solve the problems of power transmission loss, heating of electronic devices, and new green transmission magnetic suspension technology.
Main research institutes (companies):
Sumitomo Japan, Bruker Germany, Chinese Academy of Sciences, etc.
Breakthrough:
After pre-forming, after being forced to deform by external conditions, it is then processed under certain conditions and restored to its original shape to realize the design and application of the material’s reversible deformation.
Development Trend:
It has great potential in space technology, medical equipment, mechano-electronic equipment and other fields.
Main research institutes (companies):
Youyan New materials, etc.
Breakthrough:
Under the action of the magnetic field, it can produce elongation or compression performance, and realize the interaction between material deformation and magnetic field.
Development Trend:
It is widely used in intelligent structural devices, shock absorption devices, energy conversion structures, high-precision motors and other fields, and has better performance than piezoelectric ceramics under some conditions.
Main research institutes (companies):
ETREMA, American, British Rare Earth Products Company, Japan Sumitomo Light Metal Company, etc.
Breakthrough:
Liquid state, combining the magnetic properties of solid magnetic materials and the fluidity of liquids.
It has characteristics and applications that traditional magnetic bulk materials do not have.
Development Trend:
It is used in the fields of magnetic seal, magnetic refrigeration, magnetic heat pump, etc., and changes the traditional sealed refrigeration and other methods.
Main research institutes (companies):
American ATA Applied Technology Corporation, Japan Panasonic, etc.
Breakthrough:
It can sense and respond to changes in the surrounding environment, and has similar biological response characteristics.
Development Trend:
The expansion-contraction cycle of smart polymer gel can be used for chemical valves, adsorption separation, sensors and memory materials.
The power provided by the cycle is used to design the “chemical engine”.
The controllability of the mesh is suitable for intelligent drug release systems and etc.
Main research institutes (companies):
American and Japanese universities.
Introduction:
The holographic film is a groundbreaking application of hologram technology. It’s a patented projection film that, for the first time internationally, allows images to be viewed directly from both the front and back, at multiple angles including 360 degrees, regardless of the lighting conditions.
The holographic film offers dynamic aerial displays with crystal-clear imaging, while allowing viewers to see through the film to the background. It can be paired with interactive software to create three-dimensional interactive images, immersing the audience in a captivating spatial experience.
With unmatched advantages such as high definition, resistance to bright light, ultra-thinness, and anti-aging properties, it is poised to become one of the most promising materials of the future.
Future Trends:
Given its ability to provide dynamic aerial displays and clear imaging, while allowing the audience to see through to the background and interact with three-dimensional images, the holographic film presents numerous unparalleled benefits. It stands at the forefront of material innovation, destined to attract more scientific research.
Predictions for future trends in holographic film development include two main aspects:
First, molecular-level nano-optical components, centered around Holographic Color Filter Crystal (HCFC) and integrating nanotechnology with a multidisciplinary approach combining materials science, optics, and polymer science.
Second, the film will feature advanced precision optical structures within its lightweight design, ensuring superior high-definition and high-brightness imaging.
The material’s exceptional clarity and minimalist, elegant design contribute to its use in electronic devices and optical films. The development of holographic film technology is a focus for many countries, and without exaggeration, it encapsulates the future. Whichever nation masters and utilizes this technology first will lead the way into the advanced technological age.
Introduction:
Metallic hydrogen is a conductive state of liquid or solid hydrogen formed under pressures of millions of atmospheres. Its electrical conductivity is similar to that of metals, hence the name metallic hydrogen. As a high-density, high-energy storage material, metallic hydrogen was previously predicted to be a room-temperature superconductor.
It contains a tremendous amount of energy, 30-40 times greater than that of conventional TNT explosives. On January 26, 2017, the journal Science reported that Harvard University’s laboratory had successfully created metallic hydrogen. However, on February 22, 2017, due to a mishandling error, the world’s only sample of metallic hydrogen disappeared.
Theoretically, obtaining metallic hydrogen under extremely high pressure is certainly possible, yet further research is necessary for scientists to acquire samples. Most known superconductors require cooling with liquid helium (-269°C) or liquid nitrogen (-196°C), which limits the development of superconducting technology.
Unlike chemists, astronomers refer to all elements except hydrogen and helium as metals. Under high-temperature and high-pressure conditions, gaseous hydrogen can also become conductive metallic hydrogen.
For instance, Jupiter’s outer layer consists of 1000 kilometers of gaseous molecular hydrogen, beneath which lies a 24,000-kilometer layer of liquid molecular hydrogen, followed by a 45,000-kilometer layer of liquid metallic hydrogen.
In 1936, American scientist Wigner first calculated the pressure at which hydrogen transitions into a metal, suggesting that the critical pressure for this transformation ranged from one to ten million atmospheres.
Future Development Trends:
The superconducting critical temperature of metallic hydrogen, which is the maximum temperature at which it exhibits superconductivity, ranges from -223°C to -73°C. It could potentially be used at temperatures around that of solid carbon dioxide (-78.45°C), which would significantly advance superconducting technology.
Because metallic hydrogen is a high-density material, using it as a fuel would greatly reduce the size and weight of rockets, leading to a monumental leap in space exploration. The advent of metallic hydrogen, akin to the birth of the steam engine, will spark a revolutionary era in the field of science and technology.
Metallic hydrogen exists in a metastable state and could be used to create “magnetic cages” to confine plasma, containing the scorching ionized gas. Controlled nuclear fusion reactions would then convert nuclear energy into electrical energy, offering a cheap and clean power source. Such energy would enable the construction of “factories that mimic the sun” on Earth, ultimately solving humanity’s energy crisis.
Overview: A supersolid is actually akin to a superfluid, denoting a solid substance that possesses superfluid properties, essentially combining the characteristics of “superfluid + solid.” In simple terms, a supersolid not only maintains the ordered atomic arrangement typical of a crystalline state but also flows without friction, like a superfluid.
At extremely low temperatures, the vacancies within a supersolid’s crystal structure can cluster and flow freely throughout the material. If a solid object is placed within the vacancies on one side of the supersolid, it will traverse the supersolid with these vacancies, moving as freely as if it were passing through walls.
Future Trends: This novel state of matter can only exist under conditions of extreme cold and ultra-high vacuum, indicating that, for the time being, we cannot widely apply it. However, a deeper understanding of this seemingly paradoxical state of matter could enhance our comprehension of the properties of superfluids and superconductors, thus significantly advancing industries like superconducting magnets, superconducting sensors, and energy transmission.
In the future, vacancies in a supersolid will become coherent entities that can move unimpeded within the remaining solid, similar to a superfluid. The Bose-Einstein condensate is a peculiar state of matter that occurs at ultra-cold temperatures, where the quantum properties of atoms become extremely pronounced, exhibiting significant wave-like behavior.
Overview:
The wood sponge, created by chemically treating wood to strip away hemicellulose and lignin, excels in absorbing oils from water. It can soak up to 16-46 times its own weight in oil and can be reused up to 10 times. This innovative sponge surpasses all other sponges and absorbents currently in use in terms of capacity, quality, and reusability.
Future Development:
Oil and chemical spills have wreaked unprecedented havoc on water bodies worldwide. As an eco-friendly solution for cleaning up the oceans, the wood sponge presents an effective means to address this issue.
Overview:
Time crystals, also known as space-time crystals, are four-dimensional crystals that exhibit periodic structures in both space and time. Typically, we encounter three fundamental states of matter: solid, liquid, and gas.
However, as science has advanced, the concept of states of matter has expanded to include plasma, Bose-Einstein condensates, supercritical fluids, and more. Time crystals represent a novel state of matter and a non-equilibrium phase that breaks temporal translational symmetry.
The concept of time crystals was first proposed by Nobel laureate Frank Wilczek in 2012. We are familiar with three-dimensional crystals, such as ice and diamonds—geometrically symmetrical structures created by the periodic arrangement of microscopic particles in space.
While teaching his students, Wilczek pondered whether the concept of three-dimensional crystals could be extended into the four-dimensional realm of space-time, allowing matter to exhibit periodic arrangements over time.
That is to say, time crystals change states at different times, and these changes are cyclical. For example, a time crystal might be sugar at one second, brown sugar at the next, and then revert back to sugar in the third second.
Future Trends:
In September 2021, four theoretical scientists—Norman Yao, Vedika Khemani, Dominic Else, and Masaki Watanabe—were jointly awarded the “Breakthrough Prize in Fundamental Physics,” marking broader recognition for the new field of discrete time crystals.
By the end of 2021, the discrete time crystal experiment conducted by Google’s quantum computing team was named one of the year’s top physics breakthroughs by the American Physical Society (APS) Physics and the Institute of Physics (IOP) Physics World.
Research on discrete time crystals has revolutionized our understanding of periodically driven systems, many-body localization, prethermalization, and quantum thermalization processes. It has also encouraged a wide range of researchers from various fields to delve into this area.
The evolution of discrete time crystals shows that scientific exploration is often challenging, requiring refutations and rigorous academic debates. In the realm of scientific discovery, insightful errors are more valuable than mediocre truths, as they may harbor new ideas.
Time crystals have benefitted from the rapid advancements in quantum computing technology, which has allowed for their swift development rather than obscurity.
Introduction:
The Canadian biotechnology company Hyperstealth Biotechnology has developed an advanced material known as “Quantum Stealth” (Invisible Fabric). This fabric, dubbed the “Quantum Stealth camouflage,” achieves invisibility by bending light waves.
Future Trends:
This material could be used to create invisibility cloaks, aiding soldiers on the battlefield to carry out highly challenging missions through concealment. The company’s CEO, Guy Cramer, stated: “The ‘Quantum Stealth’ material can not only help special forces carry out raids during daylight but can also facilitate a soldier’s escape when they encounter unexpected danger.
Moreover, this material holds the promise for application in the next generation of stealth aircraft, submarines, and tanks, enabling them to achieve true invisibility and allowing troops to strike the enemy unseen.”
Overview: This material, composed of polymers and water, is conductive and remains perpetually moist.
Future Outlook: In the future, this material has the potential to be utilized in the creation of artificial skin and flexible robots with biomimetic capabilities.
Introduction:
Transition Metal Dichalcogenides (TMDCs) possess a simple bidimensional structure and are super-innovative materials on par with graphene. They are typically composed of a transition metal element M (such as molybdenum, tungsten, niobium, rhenium, titanium, etc.) and a chalcogen element X (such as sulfur, selenium, tellurium, etc.).
Due to their relatively low cost and ease of fabrication into exceedingly thin and stable layers, along with their semiconductive properties, TMDCs have emerged as ideal materials in the field of optoelectronics.
Future Development Trends:
If electrons and holes are injected into TMDCs, they will recombine when they meet and then emit photons. This capacity for photonic-electronic conversion holds promise for TMDCs in the domain of optical information transmission, where they could serve as miniature, low-power light sources or lasers.
TMDCs can also be combined with various two-dimensional materials to create heterojunctions with minimal lattice mismatch issues. Such heterojunction photonic devices are expected to demonstrate superior performance across a broader spectral range.
Overview:
Cryogenic boiling materials are substances that behave oppositely to thermal boiling materials, transitioning from solid to liquid to gas as the temperature decreases. These materials remain solid at high and ambient temperatures, with their strength increasing as the temperature rises, capable of withstanding temperatures exceeding 10,000 degrees Celsius.
They liquefy at -121°C and turn into gas at -270°C. Cryogenic boiling materials are considered supermaterials. Compared to the most advanced high-temperature resistant and superconducting materials currently under development, they exhibit superior high-temperature resistance and superconductivity.
When doped with inert thermal boiling materials, the low-temperature and ultralow-temperature strength of cryogenic boiling materials can be enhanced, providing exceptional strength over a broader temperature range.
Cryogenic metal materials exhibit superconducting properties at room temperature, eliminating the need for high-cost, low-temperature environments. Therefore, they hold immense potential for research and practical applications.
Future Development Trends:
Industry analysts suggest that cryogenic boiling materials could find widespread use in aerospace, supermachinery, and electronic devices. For example, in aerospace, these materials could be used to manufacture superior performance engines and spacecraft shells.
They are ideal for spacecraft that travel at the third cosmic velocity or higher, where components must maintain ultra-high hardness under extreme temperatures generated by high-velocity travel, and still function effectively in the cold and ultra-cold conditions of space.
Cryogenic boiling materials could drive a technological revolution in the aerospace industry. However, their synthesis or extraction from the moon presents significant challenges, and a long road lies ahead before these materials can be applied.
Introduction:
Magnetorheological fluids, also known as magnetic fluids, ferrofluids, or simply magfluids, represent an innovative class of functional materials that combine the fluidity of liquids with the magnetic properties of solid magnets. Composed of nanometer-scale magnetic solid particles, carrier liquids, and surfactants, these stable colloidal liquids exhibit no magnetic attraction at rest.
However, they show magnetic properties when exposed to an external magnetic field. Such unique characteristics have led to their broad application and significant academic value.
Magnetorheological fluids produced from nanometal and alloy powders demonstrate superior performance and are extensively used in demanding environments for magnetic fluid seals, damping systems, medical devices, sound modulation, optical displays, and magnetorheological separation processes.
Future Trends:
Recent years have seen numerous scientific breakthroughs in the application of magnetorheological materials across new frontiers such as aerospace, defense, healthcare, and transportation. As technology advances, these applications are expanding, and the demand for related scientific and technical expertise is steadily increasing.
Recognized as one of the materials with the most potential for future development, magnetorheological fluids have garnered international attention.
Although China entered the field of magnetorheological research later than others, it is rapidly gaining momentum. With the monopoly on high-end application technologies once held by developed countries like the UK and the USA gradually diminishing, it is anticipated that the competition in magnetorheological material research will intensify in the years to come.
Introduction:
This coating material is an iron-based, glassy alloy specifically designed for industrial drills and boring tools, offering increased resistance to fracture under heavy loads. It is significantly more cost-effective than conventional materials such as tungsten carbide-cobalt hard alloys and also enhances tunneling efficiency due to its extended lifespan.
Future Trends:
This material has potential applications in industries such as manufacturing and construction in the future.
Overview:
Perovskite nanodots, known for their colossal magnetoresistance, high ionic conductivity, electrocatalytic properties, and redox activity, hold vast potential for applications in light absorption, storage, catalysis, and sensing.
Perovskites are crystalline structural materials and represent a new class of functional materials. Currently, their stability issues are a significant barrier to development. However, research into novel perovskite structures is advancing, drawing considerable attention to perovskite nanodots.
Future Trends:
According to the “China Perovskite Nanodot Market Development Status and Industry Outlook Forecast Research Report” released by the market survey network, a team from the Queensland University of Technology (QUT) in Australia is integrating perovskite nanodots, made from human hair, into solar cells.
These nanodots form a protective layer on the surface of the perovskite, shielding the material from various external factors, enhancing its stability, and increasing the photovoltaic conversion efficiency. This can also reduce production costs.
Such advancements are crucial for the large-scale development of perovskite solar cells, indicating a promising future for perovskite nanodots.
Introduction:
Micro-lattice metals are constructed from tiny hollow tubes interconnected to form a structure, with each tube’s diameter at approximately 100 micrometers and a wall thickness of just 100 nanometers. Due to its hollow nature, the metal’s interior is comprised of 99.99% air.
This metal is primarily made up of lightweight air, allowing it to rest upon a dandelion or float to the ground like a feather from a height. Many might question the strength of such a light metal, suspecting it to be extremely fragile. However, this is not the case. Micro-lattice metals are exceptionally strong and possess a high level of compressive strength.
Future Development Trends:
As battery electrodes and catalyst carriers, micro-lattice metals are set to revolutionize the future of aviation and spacecraft manufacturing. They promise to reduce the mass of NASA’s deep-space exploration vehicles by 40%, which is crucial for future missions to Mars and beyond.
Overview:
Stanene, also known as a single layer of tin atoms, features a two-dimensional honeycomb structure similar to graphene, making it a novel quantum material. Its crystal structure is based on the diamond-like alpha-tin, and due to its non-layered configuration, it cannot be produced through mechanical exfoliation, resulting in extremely high production technology barriers.
Compared to other two-dimensional materials such as graphene, silicene, and germanene, stanene has longer bonds and superior electrical conductivity, and is poised to become the world’s first super material capable of achieving 100.0% electrical conductivity at room temperature.
Future Trends:
Industry analysts indicate that as an emerging two-dimensional material, stanene has a broad application prospect. With continuous innovation and breakthroughs in research and development technology, the range of stanene applications is expected to expand, and the industry is likely to achieve commercial development.
The technical barriers in the stanene industry are high, and in recent years, numerous Chinese research teams have made significant progress in the study of stanene materials, positively impacting the industry’s growth.
Overview:
Molecular superglue is an adhesive discovered in 2013 by Mark Howarth and his research team from the Department of Biochemistry at the University of Oxford, derived from proteins released by the bacterium Streptococcus pyogenes during cell invasion.
Inspired by the proteins emitted by Streptococcus pyogenes, the adhesive is formed from two protein components that can separate but re-bind like glue upon contact. Known as molecular superglue, this adhesive boasts high bonding strength, excellent tolerance to extreme temperatures, and resilience in acidic and other harsh environments.
Future Prospects:
Looking ahead, this material has potential applications in cancer diagnostics; molecular superglue can bond with metals, plastics, and various other substances, overcoming the common issue of poor adhesion between conventional coatings and metals.
Introduction:
Metamaterials, a term that emerged in the 21st century, refer to special composite materials or structures that possess extraordinary physical properties not found in conventional materials. This is achieved through ordered structural design of key physical dimensions.
Metamaterials intersect with numerous disciplines such as physics, chemistry, optoelectronics, materials science, semiconductor science, and equipment manufacturing, positioning them at the forefront of global research with strategic significance.
The prestigious journal Science listed metamaterials as one of the top ten scientific advancements in the first decade of this century, while Materials Today magazine also hailed them as one of the ten most significant breakthroughs in materials science over the past fifty years.
Future Trends:
Metamaterials are poised to become a new material with boundless potential. However, they are still some distance away from true large-scale industrialization, with many challenges yet to be overcome. These challenges will direct the mainstream research in metamaterials, potentially leading to further technological breakthroughs and achievements in the field.
Overview:
Quantum is an essential concept in modern physics, representing the smallest fundamental unit of matter and energy. Quantum metal is a metal comprised of the smallest particle units and is a unique two-dimensional material. It possesses the characteristics of ordinary metals, as well as insulating and superconducting properties.
Under moderate magnetic fields, it behaves as a quantum metal, transitions into an insulator under strong magnetic fields, and becomes a superconductor below -272°C. This demonstrates the potential for research into the dual-dimensional states of quantum metals.
Future Trends:
Industry analysts indicate that superconductivity is a significant direction for quantum metal research. Superconductors, which exhibit zero electrical resistance below their critical temperature, can transmit electricity without loss and have broad applications in electronics, telecommunications, power, transportation, medical, nuclear industries, aerospace, and more.
In 2021, the global superconductor market was valued at approximately $7.6 billion and continues to show a growth trend. Superconductors can be categorized into low-temperature and high-temperature superconductors, with the former holding a dominant position and strong development momentum. Quantum metal, as a type of low-temperature superconductor, holds substantial value in research and application.
Introduction:
Boron graphene, a two-dimensional material, is a single-layer planar atomic structure similar to graphene, comprised of the element boron. This thin film is just one atom thick.
Synthesized artificially, boron graphene is predicted to have a variety of structures, possessing unique properties and displaying many metallic characteristics, particularly exceptional electronic properties. It represents a new class of two-dimensional materials.
Future Trends:
Industry analysts suggest that to drive technological advancements in industry, there is a high level of interest in the research and application of new materials in the global market.
Investment from governments and capital in new material research is continuously increasing, and high-performance new materials are being developed and commercialized at an accelerated pace. As a novel two-dimensional material, boron graphene has excellent electronic properties and tremendous potential for growth in industries such as electronics and energy.
In the short term, its application market has not yet formed due to it still being in the research phase. However, in the long term, compared to graphene, it has significant market potential.
Overview:
Programmable cement, by managing the microstructure of cement particles, programs these particles to form specialized concrete with high density and low porosity. This enhances the concrete’s strength, waterproofing, and corrosion resistance.
Programmable cement is a high-tech, innovative type of cement. Not only does it boast improved overall performance, but it also significantly reduces environmental harm during production and application.
Future Development Trends:
Industry analysts indicate that China currently has lower engagement in programmable cement research compared to the United States. However, as the country aims to transition from a manufacturing giant to a manufacturing powerhouse and achieve carbon neutrality and sustainable development goals, the Chinese government is actively promoting research into high-performance, environmentally-friendly new materials.
In the future, investments in research on novel building materials in China are expected to increase steadily, and achievements in programmable cement research are likely to grow.
Overview:
Ultra-Thin Platinum is a novel method for rapidly and inexpensively depositing platinum thin films, significantly reducing the quantity of metal required for fuel cell catalysts, thereby greatly lowering their cost.
Future Outlook:
This material may be employed in fields such as hydrogen fuel cells in the future.
Overview:
Platinum alloys are composed of platinum mixed with other metals such as palladium, rhodium, yttrium, ruthenium, cobalt, osmium, and copper. As functional materials, they are used in temperature measurement, as catalysts, for electrical contacts, electrode materials, elastic materials, and magneto-hydrodynamic materials.
Platinum alloys for temperature measurement exhibit high thermal-electric stability and precision at high temperatures, primarily involving platinum-rhodium, platinum-molybdenum, and platinum-cobalt systems. Platinum-rhodium alloys have excellent high-temperature oxidation resistance and chemical stability.
Platinum-molybdenum alloy thermocouples are used for high-temperature measurements in vacuum or inert atmospheres and nuclear fields. Platinum-cobalt alloys, used in resistance thermometers, perform with high precision and sensitivity above 20K.
Platinum alloy catalysts are the sole material used in the ammonia oxidation process for nitric acid production, primarily consisting of platinum-rhodium or platinum-rhodium-palladium alloy meshes.
Future Trends:
Platinum alloys have a wide range of applications in high-temperature strain materials, precision potentiometer winding materials, medical materials, jewelry, and currency, with significant potential for future development.
Overview:
Self-healing materials, as the name implies, are capable of automatically repairing damage without the need for significant intervention. This property not only extends the lifespan of the items but also ensures their safety and integrity while reducing maintenance costs.
Research into self-healing materials began in the 1990s in the field of architectural concrete. It was not until 2001, however, that significant progress was made when the world-renowned electrochemist, American Scott White, and his team published an article in Nature. They developed polymer self-healing materials by embedding microcapsules filled with healing agents into an epoxy resin containing a catalyst, drawing widespread international attention to the field.
Future Trends:
With the rapid advancement of self-healing technology, a variety of self-healing materials are poised to find broader applications in industries such as construction, automotive, aerospace, aviation, and electronics. Their use is of great significance for resource conservation and the achievement of sustainable development.
Overview:
This innovative coating can self-adjust the transparency of glass. At temperatures above 67ºC, this transparent coating transforms into a reflective surface with a mirror-like finish to deflect sunlight.
Future Prospects:
This material has potential applications in construction, transportation, and other sectors.
Overview:
Biomimetic materials are developed to emulate various characteristics or features of living organisms. Artificial materials designed and manufactured to mimic the operational modes of living systems and the structural principles of biological materials are known as biomimetic materials.
Biomimetic plastics not only possess strength far superior to that of engineering plastics but also exhibit remarkable toughness and resistance to crack propagation. They experience minimal dimensional changes within a temperature range of -130°C to 150°C, and at room temperature, their coefficient of thermal expansion is only about one-tenth that of conventional plastics.
Future Trends:
As urbanization in our country accelerates, issues concerning social stability and urban security are increasingly coming to the fore. Biomimetic plastic technology is a key enabler for infrastructure development. Hence, with the further advancement of socio-economic and information technology, the application of biomimetic plastics is poised to become a new trend in the future.
Introduction:
Photonic crystals are periodic optical nanostructures that affect the movement of photons in much the same way that ionic lattices influence electrons in solids. They occur naturally, manifesting as structural coloration and animal reflectors, and hold promise for a variety of applications in different forms. As attractive optical materials, photonic crystals are used to control and manipulate the flow of light.
Future Trends:
One-dimensional photonic crystals are already widely utilized in thin-film optics, with applications ranging from anti-reflective and high-reflective coatings on lenses and mirrors to color-changing paints and inks. Higher-dimensional photonic crystals are of great interest for both fundamental and applied research, with two-dimensional structures beginning to find commercial applications.
Commercial products involving two-dimensional periodic photonic crystals have emerged in the form of photonic crystal fibers, which use micron-scale structures to confine light with properties fundamentally different from those guided by conventional fibers used in nonlinear devices and for guiding unusual wavelengths.
While their three-dimensional counterparts are far from commercialization, they could offer additional functionalities, such as the necessary optical nonlinearity for operating optical transistors used in optical computers, once certain technical aspects like manufacturability and major difficulties are overcome.
Overview:
Erosion-resistant ceramic materials represent a highly promising advancement in high-temperature structural materials. With a high melting point, they serve as superior refractory materials for applications such as furnaces and high-temperature kiln tubes. Among these ceramics, those categorized as structural materials are primarily composed of mechanical properties such as strength, hardness, and toughness.
While metals have been extensively used as structural materials, their susceptibility to corrosion and oxidation at high temperatures renders them unsuitable for such conditions. The advent of high-temperature structural ceramics addresses the shortcomings of weaker metallic materials. These ceramics are resistant to high temperatures, oxidation, and acid-base corrosion.
Future Trends:
High-temperature, erosion-resistant ceramics offer insulation, temperature resistance, corrosion resistance, and robust mechanical properties. High-temperature ceramic insulating coatings are recognized for their environmental friendliness, efficiency, and multifunctionality, securing an important place in the specialized coatings sector.
Overview:
Hydroceramics is a material composed of hydrogel beads that can swell up to 400 times their original volume when submerged in water.
Future Trends:
Due to this remarkable property, the spherical beads absorb liquids that will evaporate into the surrounding air during hot weather, thereby providing a cooling effect.
Overview:
Infinitely recyclable plastics refer to those that can be recycled indefinitely. Compared to conventional plastics, infinitely recyclable plastics can be reprocessed, preventing the harm caused by plastic products entering the environment, thus offering significant ecological benefits. Unlike biodegradable plastics, infinitely recyclable plastics do not degrade in nature but can be reused, providing substantial economic value.
Future Development Trends:
Infinitely recyclable plastics have a broad market outlook within the context of sustainable development strategies. Industry analysts indicate that common plastics currently face various issues concerning ecological and economic value. Infinitely recyclable plastics can address these problems to the greatest extent and replace existing plastics in the manufacture of various products.
Introduction:
The primary materials used in 4D printing are polymers. In 2014, scientists developed a tension-sensitive polymer fiber that can be fabricated into dresses capable of automatically adjusting to the wearer’s body shape and movements.
Future Trends:
Smart materials are at the heart of 4D technology. However, as research in this field is still in its infancy, there are only a few mature materials ready for market deployment, with polymers being the main focus. This presents both opportunities and challenges. A key area of current research is investigating the potential of ceramics, metals, biological substances, and composites as printing materials.
Overview:
This delicate and smooth polymer, when applied to the skin, can instantly tighten and lift, effortlessly erasing wrinkles.
Future Outlook:
This material holds great promise for development in skincare products and the treatment of skin conditions.
Below are answers to some frequently asked questions:
The latest advancements in materials science are diverse and span across various fields, reflecting significant innovations and applications. Advanced composites and smart materials are at the forefront, with new composite materials combining properties like stiffness and high damping capacity, which help reduce vibrations and noise. Smart materials, such as shape memory alloys, are being developed for adaptive applications, including aircraft wings that can respond to environmental changes.
Biomaterials and bioelectronics are also advancing rapidly. Bioelectronic materials are enhancing interfaces between electronic devices and the human body, exemplified by brain-computer interface systems like those developed by Neuralink. Additionally, new bioinks and 3D printing techniques are improving the quality and efficacy of 3D-printed organs and structures, crucial for medical research and drug testing.
In the energy sector, solid-state batteries with higher storage capacity and cost-effectiveness are being scaled up, aiding the adoption of renewable energy sources. Advances in solar energy storage and the development of high-performance, recyclable materials are also noteworthy.
3D printing technology is evolving with techniques like “Speed-Modulated Ironing,” which enables high-resolution surface textures and color gradients using a single material, reducing waste and increasing efficiency. Solvent-free 3D printing materials for biodegradable implants are being explored for medical applications.
Self-sensing and self-healing materials are making strides, with innovations that allow materials to detect strain and damage through electrical current measurements, benefiting aeroplanes, robots, and infrastructure. Self-healing materials are also being developed for healthcare applications such as electronic skin and wearable biosensors.
Nanotechnology and quantum materials are pushing the boundaries of materials science. Developments include MXene nanomaterials for wireless charging in textiles and quantum vortices confirming superfluidity in supersolids. Advances in semiconductor chip alignment and diamond spin photon quantum computers are also significant.
Medical and biomedical applications are seeing breakthroughs with synthetic helical peptides enhancing bone regeneration and hydrogel semiconductors and tissue-interfaced bioelectronics advancing bio-integrated devices and robotics.
Environmental sustainability is a key focus, with innovations in green chemistry and biologically-based bricks reducing carbon footprints. Methods for recycling micro and nanoplastics and advances in energy-efficient technologies like alkaline membrane electrolyzers for hydrogen production are also important.
These advancements demonstrate the rapid evolution of materials science, driven by interdisciplinary research and the integration of cutting-edge technologies.
New materials are significantly transforming the construction industry by enhancing sustainability, efficiency, durability, and energy efficiency. One of the key advancements is in the development of sustainable materials like biochar-based bioplastic cladding, which captures more CO2 than it emits, contributing to carbon sequestration. Similarly, Bio-Blocks and Sugarcrete offer zero-carbon alternatives to traditional concrete, reducing the industry’s carbon footprint.
Recycled materials are also gaining traction, with innovations such as recycled plastic bricks, steel, glass, and synthetic gypsum helping to minimize waste and environmental impact. Advanced structural properties are another area of improvement, with self-healing concrete that can autonomously repair cracks, extending its lifespan and reducing maintenance costs. Graphene and carbon fiber are being used to reinforce traditional materials, enhancing their strength and flexibility, while Engineered Cementitious Composite (ECC) provides a bendable, durable alternative to conventional concrete.
Energy efficiency is improved through materials like water-filled glass, which absorbs and redistributes heat, and translucent wood, which offers excellent thermal conductivity and strength. Hempcrete, a bio-composite material, provides good insulation and moisture handling, contributing to energy-efficient building designs.
Innovative construction techniques, such as 3D printing, enable the rapid construction of complex structures with precision and minimal waste. This includes the use of various materials, including metals, concrete, and polymers. Additionally, 3D-printed mycelium and living buildings are revolutionizing sustainable construction by creating self-sustaining ecosystems and utilizing urban waste as feedstock.
Waste reduction and upcycling are also being addressed with pollution-absorbing bricks that filter air and cigarette butt bricks that reduce waste and energy expenditure. Gent Waste Bricks, made from local construction waste, exemplify how recycled materials can be upcycled into durable construction elements with reduced embodied carbon.
Finally, enhanced performance and safety are achieved through innovations like light-generating cement, which emits light in the dark for energy-efficient applications, and memory steel, which reverts to its original shape when heated, making it suitable for reinforcing structures in earthquake-prone areas.
These advancements in new materials are not only making construction more environmentally friendly but also improving the structural integrity, durability, and overall performance of buildings, marking a significant shift towards a more sustainable and technologically advanced construction industry.
Several innovative materials are contributing significantly to energy efficiency and sustainability across various sectors. Sand batteries, for example, are emerging as a sustainable solution for energy storage. These batteries use low-grade sand to store thermal energy, which can then be used for heating and hot water, presenting a low environmental impact alternative to traditional chemical batteries.
In the transportation sector, rechargeable tyres are being developed for electric vehicles. These tyres feature a biodegradable tread compound that can be refilled, reducing waste and enhancing durability.
In building construction, advanced thermal insulation materials and phase change materials are being utilized to improve temperature control and reduce the need for heating and cooling. Additionally, sustainable building materials like engineered wood, recycled metal, and low-VOC paints are becoming more popular, reducing resource consumption and waste.
Next-generation materials like advanced composites, lightweight materials, and nanomaterials are also making significant strides in energy efficiency. These materials enhance the performance of energy production and transfer equipment, and nanostructured coatings are being developed for applications in energy storage and water treatment.
Efforts to develop low-cost and sustainable materials, such as alternative carbon fiber precursors and advanced battery materials, are helping to reduce the cost of renewable energy systems while improving their efficiency.
Overall, these innovative materials are pivotal in promoting energy efficiency and sustainability, addressing both environmental and economic challenges.
Advanced materials are transforming various industrial applications due to their superior properties and innovative uses. In the energy sector, advanced materials are crucial for enhancing renewable energy conversion and storage, such as through the development of sodium-ion batteries that reduce reliance on critical raw materials. The mobility sector benefits from advanced composites like carbon fiber reinforced polymers (CFRPs), which reduce the weight and improve the fuel efficiency of vehicles and aircraft, exemplified by the Boeing 787 Dreamliner and modern electric vehicles. In construction, bio-based materials and advanced composites improve energy efficiency, sustainability, and durability of buildings.
The electronics industry sees significant advancements through the use of nanomaterials, which enhance the performance and energy efficiency of integrated circuits, magnetic data storage, and other electronic components. In healthcare and life sciences, advanced materials such as nanomaterials enable innovative medical procedures and diagnostics, including imaging techniques for stem cell treatments and biosensing platforms for drug analysis.
Manufacturing and Industry 4.0 benefit from smart materials like shape memory alloys, which are used in sensors and actuators for precise control and automation. The aerospace industry adopts advanced composites to produce lighter, more fuel-efficient aircraft, replacing traditional aluminum structures. Similarly, the automotive sector uses nanocomposites and advanced composites to manufacture lightweight, strong components that improve vehicle performance and reduce emissions.
In food and consumer goods packaging, advanced materials like nanocomposites enhance barrier properties, extending the shelf life of perishable products. Lastly, industrial processes and infrastructure are revolutionized by metal additive manufacturing, which allows for precise, efficient production of intricate metal parts, and by engineered minerals like metallic magnesium alloys, which improve processes and enable new applications across various industries. These advancements drive innovation, enhance performance, and support sustainability in numerous industrial applications.
New materials have a multifaceted impact on the environment, which can be both positive and negative depending on various factors like the source, production methods, and lifecycle of the materials.
On the positive side, new materials can significantly reduce greenhouse gas emissions. For example, bio-based materials from renewable sources, such as biomass tubing made from castor seeds, help lower CO2 and GHG emissions. Innovations like “green steel,” produced using hydrogen and renewable electricity, offer sustainable alternatives to traditional high-emission materials. Additionally, new materials with high energy efficiency and biodegradability, like those developed using peptides and sustainable plastics, minimize environmental impact during both manufacturing and disposal. Furthermore, regenerative materials produced through sustainable agriculture practices help protect biodiversity and maintain soil quality.
However, there are challenges to consider. Assessing the environmental impact of new materials requires a comprehensive lifecycle analysis, from raw material extraction to end-of-life disposal, including climate impact, water usage, and effects on biodiversity. High production costs and market acceptance can also hinder the adoption of sustainable materials. Moreover, unintended consequences, such as microfiber pollution from synthetic materials, underscore the need for ongoing assessment and improvement.
Strategies to mitigate environmental impact include minimizing unnecessary extraction and production, using Environmental Product Declarations (EPDs) to make informed decisions, and adopting circular design principles to enhance recyclability and biodegradability. By evaluating the benefits and challenges of new materials and implementing these strategies, industries can promote sustainability and contribute to global decarbonization efforts.