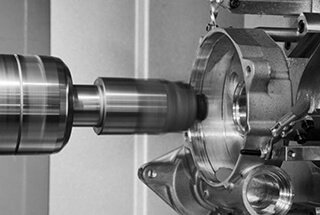
Imagine a world where cutting through the hardest materials is as easy as slicing butter. This is the promise of non-traditional machining. In this article, we explore how advanced techniques like EDM and laser machining are revolutionizing manufacturing. Get ready to uncover the secrets behind these powerful methods and their incredible applications!
First, let’s examine the definition of non-traditional machining.
Non-traditional machining, also known as “non-conventional machining” or “advanced manufacturing processes,” encompasses a diverse set of material removal and modification techniques that utilize various forms of energy. These processes harness electricity, thermal energy, photons, electrochemical reactions, chemical interactions, acoustic waves, or specialized mechanical forces to manipulate materials at the micro or macro scale.
Unlike conventional machining methods that rely primarily on mechanical cutting forces, non-traditional processes exploit unique energy-material interactions to achieve precise material removal, controlled deformation, targeted property alterations, or selective material deposition. These techniques often excel in processing difficult-to-machine materials, creating complex geometries, or achieving surface finishes beyond the capabilities of traditional methods.
The outcomes of non-traditional machining processes can include:
These advanced processes play a crucial role in modern manufacturing, enabling the production of high-precision components for aerospace, medical devices, electronics, and other cutting-edge industries.
Traditional mechanical machining has been a cornerstone of human production and material civilization for centuries. The majority of current products, from household appliances to complex transportation vehicles and defense equipment, are still manufactured and assembled using these conventional methods.
Traditional machining primarily relies on mechanical energy and cutting forces to remove excess material, shaping parts to specific geometric dimensions and surface finishes. This process necessitates that the tool material be harder than the workpiece material.
However, the rapid advancement of science and technology since the 1950s, particularly driven by demands from the defense industry, has led to increasingly complex manufacturing challenges. These include the need for high-precision, high-speed, high-temperature, and high-pressure components, as well as miniaturized products. Consequently, materials have become more difficult to machine, and product geometries more intricate, with ever-tightening dimensional tolerances and surface finish requirements.
These evolving demands have necessitated new capabilities in mechanical manufacturing, including:
To address these challenges, researchers have developed Non-Traditional Machining (NTM), also known as Non-Conventional Machining (NCM). These processes utilize various forms of energy—including electrical, magnetic, acoustic, optical, and thermal—as well as chemical energy and specialized mechanical techniques to directly affect the machining area, removing, deforming, or altering the material properties.
Key features of Non-Traditional Machining include:
These characteristics enable NTM to process a wide range of materials—both metallic and non-metallic—regardless of their hardness, strength, toughness, or brittleness. NTM excels in machining complex geometries, micro-surfaces, and low-stiffness components. Moreover, certain NTM methods are capable of achieving superfinishing, mirror finishing, and even nanometer-scale (atomic) machining precision.
As traditional machining methods reach their limits in addressing these advanced technical challenges, non-traditional machining has become an indispensable solution in modern manufacturing, continually expanding the boundaries of what is possible in material processing and product fabrication.
Non-traditional machining processes can be classified into several categories based on their energy source, functional form, and underlying principles. This classification system provides a structured approach to understanding and comparing various advanced manufacturing techniques. The following table presents a comprehensive overview of these classifications:
Machining method | Major energy form | Functional form | |
Electrical discharge machining | EDM forming machining | Electricity, Thermal energy | Melting, gasification |
EDM cutting machining | Electricity, Thermal energy | Melting, gasification | |
Electrochemical machining | Electrochemical machining | Electrochemical energy | Ion transfer |
Electroforming machining | Electrochemical energy | Ion transfer | |
Plating machining | Electrochemical energy | Ion transfer | |
High energy beam machining | Laser beam machining | Light, Thermal energy | Melting, gasification |
Electron beam machining | Electricity, Thermal energy | Melting, gasification | |
Ion beam machining | Electricity, Mechanical energy | Abscission | |
Plasma arc machining | Electricity, Thermal energy | Melting, gasification | |
Material cutting and erosion machining | Ultrasonic machining | Acoustic, mechanical energy | Abscission |
Abrasive flow machining | Mechanical energy | Abscission | |
Chemical machining | Chemical milling machining | Chemical energy | Corrosion |
Photographic plate making | Chemical energy | Corrosion | |
Light energy | |||
Lithography process | Light, Chemical energy | Photochemical, corrosion | |
Photoelectroforming plating | Light, Chemical energy | Photochemical, corrosion | |
Etching machining | Chemical energy | corrosion | |
Bonding | Chemical energy | Chemical bonds | |
Explosion machining | Chemical energy, Mechanical energy | explosion | |
Forming machining | Powder metallurgy | Thermal energy | Thermoforming |
Mechanical energy | |||
Superplastic forming | Mechanical energy | Superplastic | |
Rapid prototyping | Thermal energy | Hot melt forming | |
Mechanical energy | |||
Composite machining | Electrochemical arc machining | Electrochemical energy | Melting and gasification corrosion |
Electro-discharge mechanical grinding | Electricity, Thermal energy | Ion transfer, melting, cutting | |
Electrochemical etching | Electrochemical energy, Thermal energy | Melting, Gasification corrosion | |
Ultrasonic EDM | Sound, heat, electricity | Melting, Abscission | |
Complex electrolytic machining | Electrochemical energy, mechanical energy | Abscission | |
Cutting Base Combined Machining | Mechanical, acoustic and magnetic energy | Cutting |
This classification system allows engineers and manufacturers to select the most appropriate non-traditional machining process based on specific material properties, desired outcomes, and production requirements. Understanding these categories facilitates informed decision-making in advanced manufacturing scenarios, enabling the optimization of production processes and the achievement of complex geometries or surface finishes that are challenging or impossible with conventional machining methods.
Basic principle:
EDM, or Electro-Discharge Machining, is a type of non-traditional machining method that involves etching conductive materials through electric erosion caused by pulse discharge between two poles immersed in a working liquid. This process is also known as Discharge Machining or Electroerosion Machining. The basic equipment for this method is an Electro-Discharge Machine Tool.
Main Features of EDM:
Range of Application:
Basic Principle:
The principle of electrochemical dissolution is utilized in electrolytic machining, with the aid of a mold as the cathode. The workpiece is machined to a specific shape and size.
Range of Application:
Electrolytic machining is ideal for materials that are challenging to machine and for parts with complex shapes or thin walls.
This method has been widely used for various applications, such as gun barrel rifling, blades, integral impellers, molds, profiled holes and parts, chamfering, and deburring.
In many machining operations, electrolytic machining technology has gained a significant or even indispensable role.
Advantages:
Limitations:
Basic principles:
Laser machining is a process that uses high-energy light beams, focused by a lens, to melt or vaporize materials and remove them in a short amount of time to achieve machining.
Advantages:
Laser machining technology has advantages such as minimal material waste, cost-effectiveness in large-scale production, and versatility in machining objects. In Europe, laser technology is widely used for welding special materials such as high-grade automobile bodies, aircraft wings, and spacecraft fuselages.
Range of application:
As the most commonly used application, the technologies of laser machining mainly include laser welding, laser cutting, surface modification, laser marking, laser drilling, micro-machining and photochemical deposition, stereolithography, laser etching and so on.
Basic principles:
Electron beam machining (EBM) is the machining of materials by using the thermal or ionization effects of high energy convergent electron beam.
Main features:
High energy density, strong penetration, a wide range of one-time melting depth, large weld width ratio, fast welding speed, small thermal impact zone, small working deformation.
Range of Application:
The electron beam machining has a wide range of machinable materials and can machining on very small areas.
It achieves machining accuracy at the nanometer level, capable of molecular or atomic machining.
It has high productivity, but the cost of the machining equipment is high.
The machining process produces minimal pollution.
It is suitable for machining micro-holes and narrow slits and can also be used for welding and fine lithography.
The vacuum electron beam welding bridge shell technology is the primary application of electron beam machining in the automobile manufacturing industry.
Basic principles:
The ion beam machining is realized by accelerating and focusing the ion stream generated by the ion source to the surface of the workpiece in a vacuum state.
Main features:
Due to the precise control of ion flow density and ion energy, ultra-precision machining at the nanometer, molecular, and atomic levels can be achieved. Ion beam machining results in minimal pollution, stress, and deformation, and is adaptable to the processed materials, but comes at a high cost.
Range of application:
Ion Beam Machining can be divided into two types: etching and coating.
Etching Machining:
Ion etching is used in machining the air bearing of gyroscopes and grooves on dynamic pressure motors, with high resolution, high precision, and good repetition consistency.
Another application of ion beam etching is the etching of high-precision graphics such as integrated circuits, optoelectronic devices, and optical integrated devices.
Ion beam etching is also used for thinning materials to prepare specimens for penetrating electron microscopy.
Coating Machining:
Ion beam coating machining has two forms: sputtering deposition and ion plating.
The ionic coating can be applied to a wide range of materials. Metal or non-metal films can be plated on metal or non-metal surfaces, and various alloys, compounds, or synthetic materials, semiconductor materials, and high-melting-point materials can also be coated.
Ion beam coating technology is used for coating lubricating films, heat-resistant films, wear-resistant films, decorative films, and electrical films.
Basic principles:
Plasma arc machining is a non-traditional machining method to cut, weld and sprays metal or non-metal by the heat energy of the plasma arc.
Main features:
Range of application:
It is widely used in industrial production, especially for the welding of copper and copper alloy, titanium and titanium alloy, alloy steel, stainless steel, molybdenum used in military industry and cutting-edge industrial technology such as aerospace, such as titanium alloy missile shell, some of the aircraft thin-walled containers.
Basic principles:
Ultrasonic machining makes the surface of the workpiece gradually break by the use of ultrasonic frequency as the tool for small-amplitude vibration and punch on the processed surface by free-abrasive in the liquid between it and the workpiece.
Ultrasonic machining is often used for piercing, cutting, welding, nesting and polishing.
Main features:
Can machining any material, especially suitable for machining of various hard, brittle non-conductive material, with high precision, good surface quality, but in low productivity.
Range of application:
Ultrasonic machining is mainly used for perforation (including round holes, shaped holes and curved holes, etc.), cutting, slotting, nesting, carving of various hard and brittle materials, such as glass, quartz, ceramics, silicon, germanium, ferrite, gemstone and jade, deburring small parts in batches, polishing of mold surface and grinding wheel dressing.
Basic principles:
Chemical machining makes use of acid, alkali or salt solution to corrode or dissolve the material of the parts to obtain the desired shape, size or surface of the workpiece.
Main features:
Range of application:
RP Technology is an integration and development of modern CAD/CAM technology, laser technology, computer numerical control technology, precision servo drive technology, and new material technology. Different rapid prototyping systems have distinct forming principles and system characteristics due to varying forming materials, but the fundamental principle remains the same, which is ‘manufacturing by layers, building upon each layer.’
It is similar to a mathematical integration process, and visually, the rapid prototyping system resembles a “3D printer.
Basic principles:
The integration and development of RP technology, based on modern CAD/CAM technology, laser technology, computer numerical control technology, precision servo drive technology, and new material technology, allows for direct receipt of product design (CAD) data and the rapid manufacture of new product samples, molds, or models without the need for molds, cutters, or fixtures.
As a result, the widespread use and application of RP technology significantly shortens the development cycle of new products, reduces development costs, and improves development quality.
The transition from the traditional “elimination method” to today’s “growth method,” and from mold manufacturing to mold-free manufacturing, represents the revolutionary impact of RP technology on the manufacturing industry.
Main features:
RP technology converts complex three-dimensional machining into a series of layered machining, significantly reducing the difficulty of machining. It possesses the following traits:
The above characteristics indicate that RP technology is ideal for the development of new products, the rapid manufacture of single and small-batch parts with complex shapes, the design and production of molds and models, and the production of materials that are challenging to machine.
Additionally, it is well suited for the inspection of shape design, assembly, and rapid reverse engineering.
Range of application:
Rapid prototyping technology can be applied in the fields of aviation, aerospace, automobile, communications, medical treatment, electronics, household appliances, toys, military equipment, industrial modeling (sculpture), building model, machinery industry, etc.
In this article, we have listed nine types of non-traditional machining techniques, which could serve as a handy guide for those who want to learn about the non-traditional machining process, its advantages, classifications, and more.