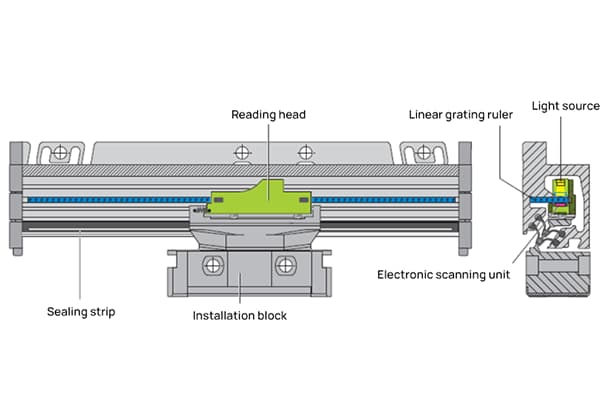
Have you ever wondered how light can turn liquid into solid almost instantly? Photosensitive resins, used in everything from 3D printing to medical devices, undergo chemical changes when exposed to UV light. This article explores their types, compositions, and how innovations are making them safer and more efficient. Dive in to understand the science behind these fascinating materials and discover their vast applications across industries.
Photosensitive resins refer to resins that undergo chemical polymerization or crosslinking upon exposure to specific light radiation, facilitated by photoinitiators, causing the monomers or oligomer base to cure. Typically, among various types of light radiation, ultraviolet (UV) radiation has activation energy closest to that required for chemical polymerization reactions.
Hence, photosensitive resins are commonly cured using UV light and are often referred to as UV-sensitive resins, UV curable resins, shadowless UV adhesives, photoresists, etc. Each photosensitive resin product has different components and responds to specific wavelengths, typically between 250-400nm.
It is important to note that UV light can be hazardous, damaging tissues and cells, and the ozone produced when it reacts with air can also affect the operating environment. Consequently, researchers are exploring photosensitive resins that cure under visible light or blue light, leading to the publication of invention patents for blue light-sensitive resins.
Photosensitive resins are primarily composed of a photosensitive pre-polymer, a photoinitiator (or photosensitizer), and a diluent.
The photosensitive pre-polymer, also known as an oligomer, is a low molecular weight pre-polymer capable of photo-curing, with a molecular weight typically between 1000-5000. It serves as the base material for photosensitive resin materials and is the decisive factor in their ultimate performance.
Main types of photosensitive pre-polymers include acrylate-modified epoxy resins, unsaturated polyesters, polyurethanes, and thiol-ene photo-curing resin systems.
Photoinitiators and photosensitizers both promote the initiation of polymerization during the curing process, yet they differ significantly. Photoinitiators participate in the reaction by creating active species such as free radicals or cations upon absorbing light energy, and are consumed in the process. Photosensitizers act more like catalysts, transferring energy without being consumed.
Photoinitiators can be classified into three categories based on their initiation mechanism: free radical type, cationic type, and hybrid type (incorporating both mechanisms). A typical free radical photoinitiator is 2-hydroxy-2-methyl-1-phenyl-1-propanone (CAS-1173), and common cationic photoinitiators include ferrocenium and iodinium salts.
The mechanism of photosensitizers involves energy transfer, hydrogen abstraction, and charge transfer complex formation. Key photosensitizers include benzoin, Michler’s ketone, thioxanthone, and benzophenone derivatives.
Reactive diluents primarily refer to low molecular weight epoxy compounds containing epoxy groups that can participate in the curing reaction of epoxy resins, becoming part of the cross-linked network structure of the cured epoxy.
Based on the number of reactive functional groups per molecule, reactive diluents can be categorized into monofunctional, difunctional, and polyfunctional diluents.
Examples include monofunctional diluents such as styrene (St), N-vinyl pyrrolidone (NVP), vinyl acetate (VA), butyl acrylate (BA), 2-ethylhexyl acrylate (EHA), and hydroxyethyl (meth)acrylate (HEA, HEMA, HPA); difunctional diluents like 1,6-hexanediol diacrylate (HDDA), tripropylene glycol diacrylate (TPGDA), and neopentyl glycol diacrylate (NPGDA); and the polyfunctional diluent trimethylolpropane triacrylate (TMPTA), among others.
Generally, the higher the number of functional groups in a diluent, the faster the rate of photopolymerization, the higher the degree of cross-linking, the better the hardness and wear resistance, but the greater the shrinkage rate. The types of functional groups mainly include acryloyloxy, methacryloyloxy, vinyl, and allyl, with the reactivity in photopolymerization decreasing in the order of: acryloyloxy > methacryloyloxy > vinyl > allyl.
The activation energy produced by irradiation can cause the C=C bonds in the photosensitive prepolymers (monomers or oligomers) to break, forming functional groups. Simultaneously, it can induce the radicals in the photoinitiators to undergo chemical polymerization or cross-linking reactions with the aforementioned functional groups.
As a result, the resin matrix’s small molecular chains are woven into larger molecular chains or even three-dimensional networked molecular chains, as illustrated in Figure 4-63. The resin thus transitions from a liquid to a solid state. It should be noted that oxygen generally hinders the aforementioned polymerization or cross-linking reactions in most photosensitive resin matrices.
The CLIP process effectively exploits this characteristic to prevent the resin from curing against the vat.
Photosensitive resins can be classified into different types based on various categorization methods.
Based on the solvent used, photosensitive resins can be divided into solvent-based and water-based categories. Solvent-based photosensitive resins are hydrophobic and can only be dissolved in organic solvents, not in water.
Common solvent-based photosensitive resins include UV polyether acrylates. Water-based photosensitive resins are hydrophilic, capable of being decomposed or dispersed in water. These resins contain a certain number of hydrophilic groups and unsaturated groups, which make water-based photosensitive resins hydrophilic, like water-based polyurethane acrylates.
Transparent Photopolymer Resin: This resin is naturally transparent and can be polished to a semi-transparent or fully transparent finish. It is primarily used for visual and structural verification of various products, allowing for highly detailed and cost-effective surface finishes.
Solid Color Photopolymer Resin: The resin’s natural color is solid, and its surface can be polished, painted, or electroplated. It is mainly used for structural verification of products, enabling extremely fine surface finishes at the highest cost-effectiveness.
High-Temperature Photopolymer Resin: The resin’s natural color is solid and is mainly used for products that require a certain level of high-temperature resistance. It can withstand temperatures up to 100-110°C, slightly higher than standard photopolymers.
High Toughness Photopolymer Resin: Typically yellow-green in color, this resin has slightly higher toughness than standard photopolymers, allowing for slight bending.
In the realm of desktop 3D printers, fused deposition modeling (FDM) printers currently dominate in terms of price and versatility, gaining widespread popularity both domestically and internationally.
However, when higher precision and better surface details are required, low-cost Stereolithography (SLA) and Digital Light Processing (DLP) 3D printers have a clear advantage. The increasing availability of affordable SLA and DLP 3D printers has spurred the evolution of photopolymer material technology.
General Purpose Resin: Initially, 3D printing resin manufacturers sold their proprietary materials, but as market demand grew, numerous resin manufacturers emerged, including MadeSolid, MakerJuice, and Spot-A. Initially, desktop resins were limited in color and performance, with materials typically available only in yellow and transparent.
Recent developments have expanded the color options to include orange, green, red, yellow, blue, white, and more.
Rigid Resin: Photopolymer resins used in desktop 3D printers tend to be fragile, prone to breaking and cracking. To address these issues, many companies have started producing more robust and durable resins.
For example, Formlabs introduced a new Tough Resin material that strikes a balance between strength and elongation, giving 3D printed prototypes better impact resistance and strength. This is particularly useful for prototypes of precision components or snap-fit connectors.
Castable Resin for Investment Casting: Traditional investment casting processes can be complex and lengthy, and mold constraints often limit design freedom. This is especially true when compared to 3D printed wax patterns, which do not require mold-making for the wax models.
Castable resins exhibit low expansion and require complete polymer burnout during the casting process to leave a flawless final product shape, as any plastic residue can cause defects and deformation in the cast. Equipment manufacturers like SprintRay and specialized material producers such as Fun ToDo offer such resins.
Domestic company Su-Cheng Technology has also launched CA resin for investment casting. Figure 4-64 shows some investment casting models made with this type of resin.
Flexible Resin: Manufacturers of flexible resins include Formlabs, FSL3D, Spot-A, Carbon, and Su-Cheng Technology. These resins have a medium hardness and are wear-resistant, capable of being stretched repeatedly. This material is used for parts such as hinges, friction devices, and components that require repeated stretching. Figure 4-65 displays models made from flexible resin.
Elastic resin is a material that exhibits excellent elasticity under high-strength extrusion and repeated tension. Formlabs’ Flexible resin is a very soft rubber-like material that becomes quite pliable when printed with thinner layers and highly elastic and impact-resistant with thicker layers. Its potential applications are boundless.
This novel material is poised to revolutionize the manufacturing of perfect hinges, dampers, contact surfaces, etc., catering to those with imaginative ideas and designs. Figure 4-66 shows a model made of elastic resin.
High-temperature resin is undoubtedly a focal point of research and development among many resin manufacturers. This is because the aging issue of these plastics has long been a challenge in progressing resin from consumer to industrial applications. Cyanate ester resin boasts a heat deflection temperature of up to 219°C, maintaining good strength, stiffness, and long-term thermal stability at high temperatures.
It is ideal for automotive and aerospace industry molds and mechanical parts. The current challenge for high-temperature resin materials is achieving a heat deflection temperature (HDT) of up to 289°C (552°F). Formlabs has also introduced its latest high-temperature material.
Formlabs’ Dental SG material for desktop 3D printers complies with EN-ISO10993-1:2009/AC:2010 and USP Class VI standards, ensuring safety and environmental friendliness for human tissue. Due to the resin’s translucency, it can be used in surgical materials and as guides for surgical drills. While it is designed for the dental industry, this resin is also applicable in other fields, particularly throughout the medical sector.
Ceramic created by the photopolymerization of preceramic monomers using UV light exhibits uniform shrinkage and virtually no porosity. After 3D printing, this resin can be sintered to produce dense ceramic parts. The ultrahigh-strength ceramic material produced with this technology can withstand temperatures exceeding 1700°C.
The prevalent ceramic photopolymerization techniques on the market involve dispersing ceramic powder uniformly into a photopolymerizable solution through high-speed stirring, creating a high solid content, low-viscosity ceramic slurry.
This slurry is then directly solidified layer by layer on a photopolymerization molding machine to accumulate the ceramic green body, which is subsequently dried, debound, and sintered to obtain the final ceramic parts.
Daylight resin is a fascinating type of resin that, unlike those cured under UV light, can solidify under normal daylight. This eliminates the dependency on UV light sources, allowing a liquid crystal display to be used for curing. This resin holds the promise of significantly reducing the cost of photopolymerization 3D printing and has a very promising outlook.