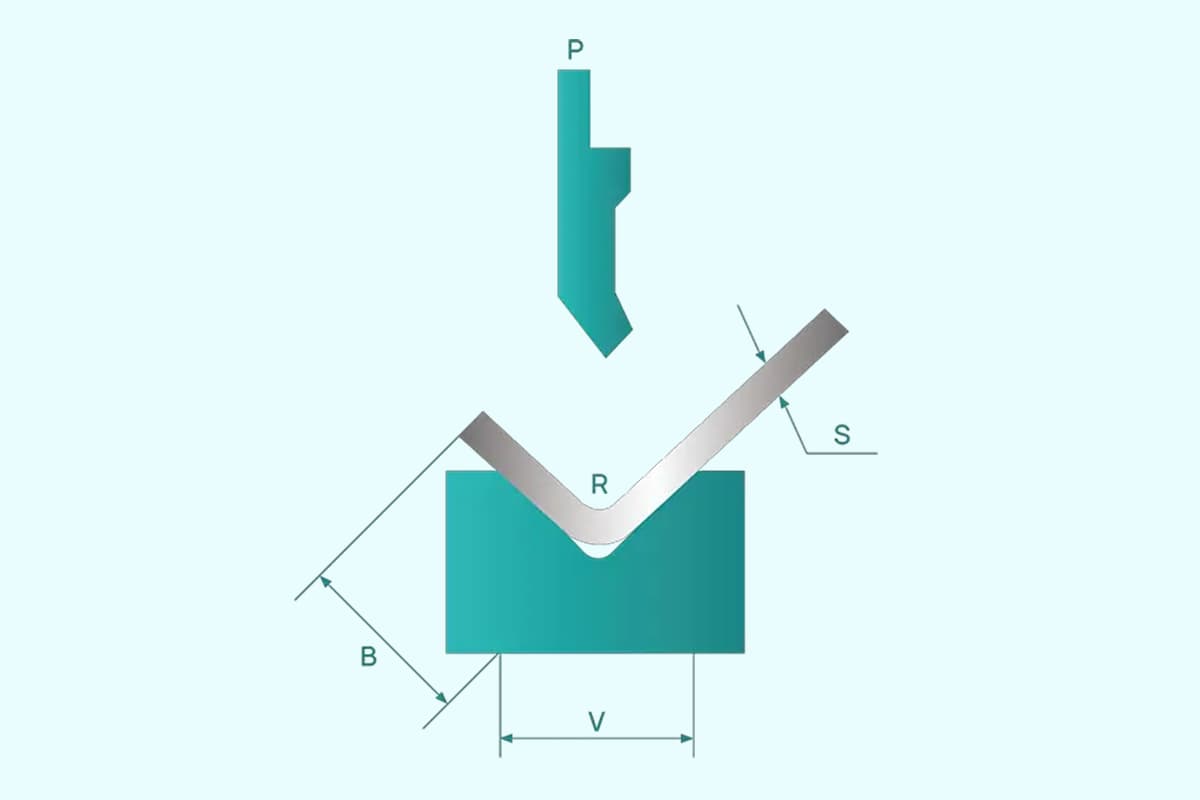
Ever wondered why your metal bends aren’t always perfect? The secret lies in press brake crowning, a technique that ensures precision and quality in metal fabrication. This article will reveal how different crowning methods can transform your bending results, enhancing accuracy and consistency. Dive in to uncover the key to flawless metalwork!
Press brake crowning is a critical process in metal fabrication that ensures the precision and straightness of bent workpieces. This technique compensates for the natural deflection that occurs during the bending process, significantly enhancing the quality and consistency of the final product.
When a metal sheet is subjected to bending forces in a press brake, the applied pressure concentrates in the center of the workpiece. This concentration causes a deflection not only in the material but also in the machine components, including the ram, worktable, and both upper and lower dies. The result is an uneven pressure distribution along the bending line, which can lead to inconsistent bend angles and compromised part quality.
To counteract this inherent deflection, engineers have developed various deflection compensation devices, with press brake crowning being one of the most effective solutions. The principle behind crowning is to introduce a calculated curvature to the bending components that precisely offsets the expected deflection during operation. This pre-emptive adjustment ensures that when the bending force is applied, the die edge remains straight and uniform along its entire length, resulting in consistently accurate bends.
There are three primary methods of press brake crowning:
Each crowning method has its own set of advantages and limitations. Factors such as the press brake’s size, typical workload, required precision, and budget all play crucial roles in determining the most suitable crowning solution for a specific manufacturing environment.
Understanding and implementing the appropriate crowning technique is essential for fabricators aiming to produce high-quality, precision-bent components. It not only improves the accuracy of bends but also extends the lifespan of tooling and reduces material waste due to inconsistencies.
For those seeking to optimize their metal fabrication processes, a deeper exploration of press brake crowning methods and their applications can lead to significant improvements in product quality, operational efficiency, and overall manufacturing capabilities.
Crowning is a sophisticated compensation system designed to counteract the inherent deformation of press brakes during bending operations. In a typical 3-meter press brake, the elastic structure of the machine can induce a deviation of up to 0.15mm along the Y-axis at the center of the ram.
To mitigate this deformation, crowning generates an opposing force that ensures uniform pressure distribution along the entire length of the metal sheet during bending. This precision control prevents common bending issues such as curved profiles and inconsistent angles.
The press brake’s ram is actuated by two working cylinders positioned at its extremities. Consequently, the bending force concentrates at the center, causing both the ram and worktable to deflect in conjunction with the upper and lower dies. This deflection results in non-uniform pressure distribution along the die edge, directly impacting the accuracy and linearity of the bent workpiece.
The crowning system, or deflection compensation device, is engineered to counteract this deformation. It is pre-calibrated to induce a deformation opposite to the force-induced deflection in the ram, upper die, worktable, and lower die. The magnitude of this compensatory deformation is precisely matched to the actual working conditions, effectively neutralizing the unwanted deflection.
By implementing crowning, the relative deformation between the ram and worktable is minimized, resulting in a more uniform pressure distribution across the dies. This enhancement significantly improves the bending quality and dimensional accuracy of the sheet metal components.
Modern press brakes often feature advanced automatic crowning systems. These can include:
In active crowning systems, sensors continuously monitor pressure fluctuations and instantaneously adjust the compensation, maintaining optimal deformation control throughout the bending process. This real-time adaptation ensures consistent bending quality across varying material thicknesses and lengths, significantly enhancing the overall precision and efficiency of press brake operations.
The primary purpose of crowning in press brake operations is to compensate for machine deflection and ensure precise, consistent bending across the entire workpiece length. By providing a calculated curvature to the bed or ram, crowning maintains uniform force distribution throughout the bending process, counteracting the natural tendency of the machine to bow under load.
Crowning plays a crucial role in press brake operations by:
Crowning methods range from manual adjustments using mechanical systems (e.g., wedges or shimming) to sophisticated CNC-controlled hydraulic or electromechanical systems. Advanced crowning technologies offer:
Implementing an effective crowning system is essential for press brake operators to achieve optimal bending accuracy, consistency, and efficiency. It not only improves part quality but also enhances productivity by reducing setup times and minimizing scrap rates, particularly in high-precision applications or when working with challenging materials.
In modern sheet metal fabrication, press brake crowning is a critical technique used to compensate for machine deflection and ensure uniform bending along the entire length of the workpiece. Currently, there are three primary types of press brake crowning systems employed in the industry:
Press brake manufacturers generally avoid this crowning method due to its limitations. The technique involves machining the working table into a convex arc shape, with a slight elevation in the center, to counteract the deflection caused during bending operations.
To complement this, the upper die is precision-ground with a subtle curvature in its middle section. This design aims to maintain a straight die edge when the ram experiences upward deflection, theoretically ensuring uniform bending force distribution along the entire bend line.
While geometric crowning offers advantages such as cost-effectiveness and relative ease of manufacturing, it presents significant drawbacks:
The primary challenge lies in the method’s inflexibility to adapt to varying material properties, thickness variations, and diverse bending scenarios. Modern manufacturing demands more dynamic compensation techniques that can adjust to real-time bending conditions.
Despite its ability to achieve some degree of deflection compensation, the practical implementation of geometric crowning proves highly challenging in today’s precision-driven metalworking industry. As a result, more advanced and adaptive crowning methods have gained prominence in contemporary press brake designs.
Hydraulic crowning is predominantly employed on electro-hydraulic synchronous CNC press brakes, where precise compensation control is essential. This method is favored due to its compatibility with advanced controllers such as DA52S, DA66T, and similar systems, allowing for dynamic and accurate adjustment during the bending process.
The system typically comprises four hydraulic cylinders: two primary cylinders positioned on either side of the press brake frame, and two auxiliary cylinders located centrally. During operation, the auxiliary cylinders are pressurized with hydraulic fluid, creating a controlled downward deflection that compensates for the natural bending of the beam under load.
An advanced variation of this system incorporates auxiliary hydraulic cylinders beneath the worktable. This configuration generates an upward force, effectively creating an automatic crowning system that can adapt to varying material properties and bending requirements in real-time.
The pressure compensation mechanism is a sophisticated assembly consisting of multiple small oil cylinders, a motherboard, an auxiliary plate, pin shafts, and a compensating cylinder integrated into the worktable. A proportional relief valve regulates the system, ensuring precise pressure control. During operation, the auxiliary plate provides support for the oil cylinders, which in turn exert just enough upward force on the motherboard to counteract the combined deformation of the ram and worktable.
The crowning device is governed by a numerical control system that calculates the optimal preload based on critical parameters such as material thickness, die opening width, and the tensile strength of the workpiece. This allows for adaptive compensation across a wide range of sheet materials and bending scenarios.
Hydraulic crowning offers significant advantages, particularly in its ability to provide continuous variable deformation compensation with high flexibility. This makes it ideal for complex bending operations and varying material thicknesses. However, it’s important to note that the system’s complexity and relatively high initial cost can be limiting factors for some applications.
To optimize the use of hydraulic crowning, manufacturers should consider:
Mechanical crowning is a highly effective and cost-efficient deflection compensation method widely adopted in conventional press brakes. Its popularity stems from its operational simplicity and user-friendly nature, making it an invaluable tool for press brake operators in real-world manufacturing environments.
This innovative compensation technique typically employs a triangular oblique wedge mechanism. The core principle revolves around the use of two triangular wedge blocks, each with a specific angle α. The upper wedge is constrained in the X-direction but allowed to move freely along the Y-axis. When the lower wedge is displaced by a distance △x along the X-axis, the upper wedge is forced upward by a height H due to the reactive force from the lower wedge.
In contemporary mechanical compensation systems, two full-length bolster plates are strategically positioned on the press brake worktable. These plates are interconnected using disc springs and precision bolts, allowing for controlled relative movement. Both the upper and lower plates incorporate a series of oblique wedges with varying slopes. This design enables a motor-driven system to induce relative motion between the plates, generating an ideal curved profile composed of multiple convex positions along the length of the brake.
The precision of this system allows for fine-tuning of the bending force distribution, effectively counteracting the natural tendency of the press brake beam to deflect under load. By adjusting the relative positions of the wedges, operators can achieve consistent bend angles across the entire workpiece, even when working with longer or thicker materials that would typically pose challenges due to beam deflection.
Crowning is a critical process in press brake operations that compensates for machine deflection during bending, ensuring consistent bend angles along the entire workpiece length. To set up the crowning system, operators input key parameters into the machine’s control system, including sheet thickness, material length, die opening, and material tensile strength. Advanced control systems utilize finite element analysis (FEA) to calculate the precise deflection of the table and ram, determining the optimal preloading required for each bend.
Three primary crowning methods are employed in modern press brakes:
Manual and semi-automated methods often involve shimming the die on the bed or adjusting mechanical wedges to correct alignment and maintain bend accuracy. These methods, while cost-effective, can be time-consuming and prone to operator error. Programmable CNC crowning systems offer superior precision and efficiency by automatically adjusting the bed or ram curvature based on the calculated deflection, significantly reducing setup time and enhancing repeatability.
Proper workpiece handling and alignment are crucial for achieving optimal bending results. The sheet metal must be carefully positioned on the press brake, ensuring it is square to the die and centered along the bending length. Many modern press brakes incorporate laser positioning systems or CNC back gauges to assist in precise workpiece alignment.
Before initiating the bending cycle, operators must verify that the crowning system is correctly adjusted for the specific job. This may involve running test bends on scrap material and measuring the resultant angles along the bend length to confirm uniformity.
During the bending process, the ram descends, applying force to the workpiece. The crowning system dynamically compensates for the machine’s deflection by adjusting the bed or ram curvature. This compensation ensures that the bending force is uniformly distributed along the entire length of the workpiece, resulting in consistent bend angles and minimizing springback variations.
For complex parts or high-precision requirements, some advanced press brakes incorporate real-time force monitoring and adaptive control systems. These systems can make minute adjustments to the crowning profile during the bending cycle, compensating for material variations or thermal expansion effects.
In summary, the crowning process in press brake operations is a sophisticated interplay between machine setup, material properties, and dynamic compensation. By leveraging advanced crowning systems and proper workpiece handling techniques, manufacturers can achieve superior bend quality, reduce scrap rates, and improve overall productivity in sheet metal forming operations.
When the press brake machine is in operation, it will cause deformation, which is mainly due to the application of force at both ends of the machine. This force, generated during the bending process, causes deformation in the ram and the worktable, resulting in inconsistencies between the two ends of the workpiece and its central angle.
To analyze the press brake machine, the finite element method is widely used due to its speed and accuracy.
Convex curve of 100 ton 3-meter press brake machine obtained by finite element method:
There are several methods to compensate for deflection deformation:
The worktable has a three-layer splint design, with compensating oil cylinders located throughout the structure.
When the system applies pressure to the compensating cylinders, it pushes up the middle splint of the three-layer splint, resulting in compensation for the deformation.
To control the position, compensation is provided at the corresponding point during bending to counteract the elastic deflection deformation of the machine.
Mechanical compensation is achieved through a group of wedges with inclined planes, which can provide reverse compensation.
Before bending loading, pre convex state
After the bending is loaded, the actual compensation state is changed
Loading simulation animation of the convex worktable
Driving mode
When it comes to bending workpieces, there are two key factors that determine its accuracy:
Fig. 1 Tx and Ty schematic
The greater the depth of the upper die of the press brake into the lower die, the smaller the bending angle.
Based on Figure 2, it can be calculated that when bending a 2mm carbon steel plate to 135° using the V12 lower die, a height direction deviation of 0.045mm can result in an angle deviation of 1.5°.
Fig. 2 Influence of height direction deviation on angle deviation
Further reading:
When a workpiece is bent using a press brake, the upper and lower beams may experience deflection and deformation due to their structural characteristics and the bending force applied, as illustrated in Figure 3.
Fig. 3 Deflection and deformation diagram of upper and lower beams
Currently, there is an inconsistency in the depth of the upper die entering the opening of the lower die along the full-length direction of the workpiece. This inconsistency can cause excessive deviation of the bending angle of the workpiece along its full-length direction.
This inconsistency typically results in a workpiece with a large middle angle and smaller angles at both ends, as depicted in Fig. 4.
Fig. 4 Schematic diagram of bending angle
Therefore, to ensure consistency of bending angle along the entire length of the workpiece, a crowning system needs to be introduced in the press brake.
As mentioned above, when the press brake bends the workpiece, the upper and lower beams, due to their structural characteristics, undergo deflection deformation under the bending force. This can lead to excessive deviation of the bending angle of the workpiece in the full-length direction.
However, the crowning system can effectively compensate for the deflection deformation of the press brake. By using the crowning system on either the upper or lower beam, the consistency of the bending angle can be ensured throughout the length of the workpiece.
The crowning system is divided into two categories:
The hydraulic crowning system operates on the principle of embedding several hydraulic cylinders in the lower beam of the press brake. Each hydraulic cylinder can be controlled separately, causing the lower beam to form a certain bulge, as illustrated in Figure 1.
Theoretically, using more hydraulic cylinders increases the number of compensation points, resulting in higher compensation accuracy.
Hydraulic crowning is a discrete compensation method that is built-in.
To achieve a high-resolution compensation effect and high bending accuracy, the number of hydraulic cylinders and their hydraulic control system must meet higher requirements, resulting in a more complex overall structure and a higher cost of the press brake.
It is not possible to retrofit the hydraulic crowning system onto an existing customer press brake.
Fig. 1 Schematic diagram of hydraulic crowning system
The mechanical crowning system utilizes the filling method to compensate for the lower beam/lower die. Its main principle involves generating various compensation curves by means of the mutual movement of a pair of deflection compensation wedges, as demonstrated in Figure 2.
Fig. 2 Schematic diagram of mechanical crowning system
There are many types of mechanical crowning systems available in the market.
Let’s take the example of Wila’s mechanical compensation workbench. It falls under the category of external, relatively continuous compensation. This system can be directly installed on the lower beam of the press brake and is suitable for both new and old press brakes.
The compensation curves of this system can be adjusted continuously for various applications, as demonstrated in Fig. 3.
Further reading:
The length of a sheet metal bend greatly affects its bending accuracy. The longer the sheet metal, the greater the bending force required, leading to larger equipment inclinations and ram deformations, making accuracy harder to ensure. This bending accuracy, including the total bending length, is referred to as “straight line accuracy.”
Without effective measures, inconsistent amounts of concave die entering the full length direction of the bending upper die can cause the bending part to have a “boat belle” effect. To address this issue, a finite element simulation method was used to analyze the ram’s force and deformation displacement. The deflection compensation curve was extracted and modified, and combined with empirical data to design and manufacture a new mechanical deflection compensation device.
The linear accuracy of large-size press brake machines can be improved by using a driving motor or manual adjustment to compensate for deflection in the whole or part of the length.
Modeling
The press brake ram is made of steel plates of various shapes. During the modeling process, only the main structure of the ram is considered, while details that have little impact on the results are ignored. The main body dimensions are 8000mm x 2500mm x 120mm.
The elastic modulus is set to 2 x 105 MPa, Poisson’s ratio to 0.27, and density to 7.8 x 103 kg/m3. Given the structural characteristics of the ram, a solid95 element defined by 20 nodes was selected for the analysis.
This element has the capability to adapt to curved boundary models and accurately analyze the elastic deformation of the ram, as it has arbitrary 3D orientation.
(1) Constraints
In real-world conditions, the ram is always in motion. However, to perform a static analysis of the ram, it is necessary to simplify and approximate the constraints of the ram. To do this, symmetrical constraints are imposed on the nodes located on the middle symmetry plane of the ram.
The ram is fixed by connecting the guide rail on the frame to its back, where a full constraint is applied. This ensures that the ram remains in a fixed position during the analysis.
(2) Load condition
The surface load is applied to the contact area between the bottom of the hydraulic cylinder and the ram block. As the vertical deformation of the ram block is small compared to its total length, it is considered to be small elastic deformation. As a result, a uniform load is applied to the stress surface at the bottom of the ram block in the model.
To ensure that the force is evenly transmitted from the ram block to the upper die, the bottom of the ram block is connected to the upper die by a connecting block. This ensures that the load is distributed evenly and does not cause any imbalances in the system.
Extraction and analysis of simulation results
The displacement diagram of the ram block under load is shown in Figure 1. The path is set in ANSYS for result processing, and the deformation deflection curve of the stress surface at the bottom of the ram is extracted and shown in Figure 2.
As seen in the figure, the maximum displacement appears at the center of the ram and decreases gradually towards both sides in a parabolic shape. At the same time, the deformation displacement at any position along the bending length direction can be obtained, providing data support for designing wedges with different array angles to form the deflection curve.
The analysis shows that when a press brake machine is loaded, its stress surface on the ram produces parabolic deflection deformation due to its own structure, resulting in inconsistent bending angles of the workpiece along its full length. Additionally, local wear on the bending die also affects the straightness of the bent workpiece.
Currently, there are two common methods to address this issue. The first method is to install a hydraulic top cylinder at an appropriate position on the upper ram or lower worktable of the bending machine and control the ejection height of each top cylinder to compensate for the deformation. The second method is to use a mechanical deflection compensation device on the lower worktable, which compensates for deformation by adjusting the wedge blocks with different angles.
The hydraulic top cylinder method is easy to operate and meets the general accuracy requirements of bending production. However, for large-size and high-accuracy bending parts, the mechanical deflection compensation method is primarily used.
The traditional method for mechanical crowning involves manual adjustment of the compensation block or adding a gasket at worn areas, which is time-consuming, labor-intensive, and not very efficient, making it difficult to guarantee accuracy.
More advanced press brake machines, on the other hand, have automatic or semi-automatic deflection compensation mechanisms, such as the commonly used wedge type and pull rod type compensation devices. The wedge type device can ensure consistent angles and improve bending accuracy, but it requires a lot of manual labor and is not very efficient. The pull rod type device, on the other hand, easily compensates for deflection along the whole length, but it does not solve the issue of local wear.
Figure 3 (a) and (b) depict two types of deflection compensation devices.
Fig. 3 Common mechanical deflection compensation device
To address the issue of local wear, our mold company designed a four-piece wedge deflection compensation device. This device not only automatically compensates for the entire deflection of the workpiece, but also allows for manual adjustment to compensate for local wear of the die.
Figure 4 is a two-dimensional sectional view of the device, and its working principle is described as follows:
Fig. 4 Four piece wedge type deflection compensation device
(1) A rectangular groove is set along the length direction (i.e. longitudinally) on the base. In this groove, odd groups of wedge mechanisms are evenly distributed longitudinally. Each group consists of two pairs of four wedges, i.e. Wedge I, Wedge II, Wedge III, and Wedge IV, stacked from bottom to top.
(2) In each group of wedges, the lower pair, Wedge I and Wedge II, form a local adjusting mechanism. The inclined planes of each pair are matched correspondingly and arranged in a transverse direction.
Screw holes are set in the middle of the front and rear seat walls of the base corresponding to the big end of Wedge I. Adjusting bolts are installed on the outside of the base wall and each one extends into the base to connect with Wedge I.
To achieve local compensation, the bolt can be adjusted manually to move Wedge I forward and backward (transversely), thereby adjusting the upper cover plate and causing the worktable to move up and down.
(3) The upper pair, Wedge III and Wedge IV, form an integral adjusting mechanism. They are set longitudinally in each group and form an integral adjusting inclined wedge device.
Each pair of Wedges III are matched with the inclined plane of Wedges IV, with the largest inclination located in the middle of the rectangular groove on the base. The inclination gradually decreases towards the left and right sides of the groove. When the Wedges III move equidistantly along the length direction, the middle lift is substantial, forming a curve that adjusts the deflection based on the movement of the Wedges. This realizes the overall deflection compensation.
The short axis of each Wedge IV is symmetrically arranged on the front and rear side walls. A vertical notch groove is arranged on the upper part of the front and rear side walls of the rectangular groove of the base, corresponding to the short axis. The short axis of each Wedge IV slides in each notch groove, allowing only up and down movement and ensuring the lifting effect of Wedge IV.
(4) Longitudinal screw holes are set on Wedge III at the right end, while longitudinal through holes with the same center line as the screw holes are set on the other Wedge III. A hollow spacer sleeve is installed between each pair of adjacent Wedge III. A pull rod is installed in each Wedge III and hollow spacer sleeve. The right end of the pull rod is threaded into the Wedge III at the right end. An adjusting screw is installed at the right part of the screw hole of the Wedge III at the right end, and a motor is installed at the end of the adjusting screw to start the motor, which can achieve automatic overall deflection compensation.
Figure 5 shows an 8-meter-long device for double pull rod four-piece wedge deflection compensation.
Fig. 5 8m double strut wedge type deflection compensation device
In this post, the small elastic deformation of the ram in a press brake machine is simulated and analyzed, and the deflection deformation data of the stress surface at the bottom of the ram is extracted.
Based on the experience data, a four-piece wedge deflection compensation device has been designed. It not only automatically adjusts the overall deflection compensation of the processed parts but also allows for manual adjustment of local die wear compensation.
The device has a well-designed structure, is convenient and reliable to use, improves the quality and production efficiency of sheet metal bending parts, and provides a new solution for large precision bending compensation.