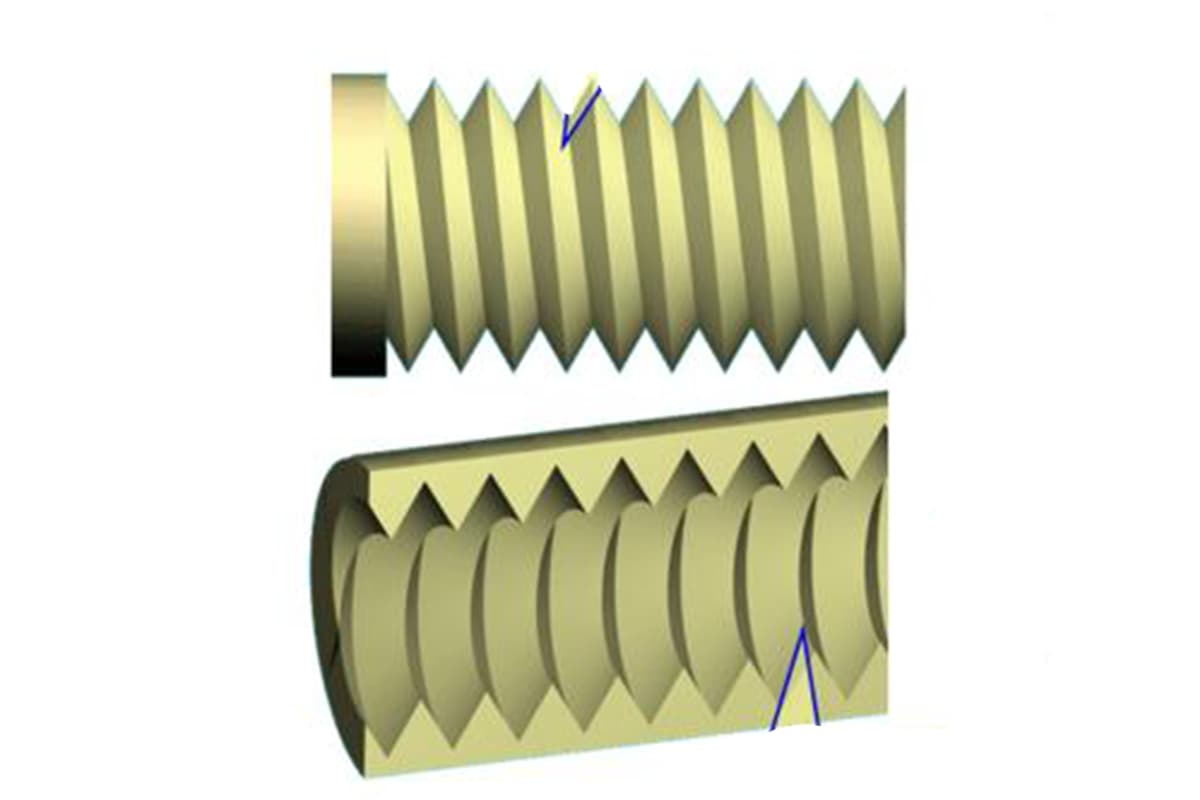
Looking for top vacuum pump manufacturers? This article highlights the leading brands in the industry for 2024, such as NASH, Edwards, Leybold, and Atlas Copco. Each company is renowned for its innovation and reliability in producing high-quality vacuum technology. Whether you need pumps for industrial applications, scientific research, or everyday products, these brands have set the standard. Dive in to discover the best manufacturers, their histories, and what makes them stand out in the market.
Vacuum refers to a space with significantly reduced gas content compared to atmospheric conditions. In practical engineering applications, a vacuum is defined as any environment where the gas pressure is lower than atmospheric pressure (101,325 Pa or 760 Torr at sea level). The degree of vacuum is characterized by the extent of this pressure reduction, ranging from low to ultra-high vacuum.
True vacuum, or absolute zero pressure, represents a theoretical state where a volume contains no matter whatsoever. However, achieving this ideal condition is practically impossible due to fundamental physical limitations, including quantum effects and the omnipresence of cosmic radiation.
In industrial processes, vacuum technology plays a crucial role in various applications, such as thin-film deposition, electron microscopy, and space simulation chambers. The level of vacuum required depends on the specific application, with some processes demanding ultra-high vacuum conditions (below 10^-7 Pa) to minimize molecular interference and contamination.
Understanding vacuum concepts is essential for optimizing manufacturing processes, particularly in industries like semiconductor fabrication, aerospace testing, and advanced materials research. Proper vacuum system design and maintenance are critical for ensuring process efficiency, product quality, and equipment longevity in these high-tech manufacturing environments.
Vacuum degree, also known as vacuum level or vacuum quality, quantifies the extent of gas removal from a confined space, typically expressed in pressure units. It represents the rarefaction of gas molecules in a given volume, with lower pressure values indicating a higher vacuum degree. This parameter is crucial in numerous industrial applications, especially those demanding precisely controlled environments free from contaminants and unwanted chemical reactions.
In metal processing and manufacturing, vacuum technology plays a pivotal role in several critical processes:
The precise control of vacuum degree is essential for optimizing these processes, as it directly impacts product quality, process efficiency, and material properties. Furthermore, in emerging technologies like additive manufacturing of metals, vacuum conditions are instrumental in preventing oxidation and ensuring the production of high-density, defect-free parts.
In industrial metal processing and manufacturing applications, two primary types of vacuum degrees are crucial:
Absolute Vacuum: This represents the theoretical total absence of any gas molecules or particles. While achieving a perfect absolute vacuum is practically impossible, it serves as a critical reference point. In metal processing, absolute pressure is measured relative to this perfect vacuum state. This measurement is essential in processes such as vacuum heat treatment, where precise control of the chamber environment is crucial for achieving specific material properties.
Relative Vacuum (Gauge Pressure): This refers to the pressure difference between the system’s internal pressure and the ambient atmospheric pressure. Often termed gauge pressure, it is the most commonly used measurement in industrial vacuum systems. Relative vacuum is particularly important in processes like vacuum forming of sheet metal, vacuum casting, and vacuum-assisted resin transfer molding (VARTM) for composites. The gauge pressure reading directly indicates the effective vacuum level, which is crucial for process control and quality assurance.
Understanding these vacuum types is essential for optimizing various metal manufacturing processes, including:
Accurate measurement and control of these vacuum types directly impact the efficiency, quality, and repeatability of advanced metal manufacturing processes.
The value displayed on a vacuum gauge is referred to as the degree of vacuum. This measurement quantifies the extent to which the actual system pressure is reduced below atmospheric pressure. The gauge pressure, in this context, represents the differential between atmospheric pressure and the absolute pressure within the vacuum system.
Understanding vacuum measurements requires consideration of several key factors:
1. Absolute vs. Gauge Pressure: While absolute pressure is measured relative to perfect vacuum (zero pressure), gauge pressure is measured relative to atmospheric pressure. In vacuum systems, gauge pressure is typically negative.
2. Units of Measurement: Vacuum can be expressed in various units, including torr, pascals (Pa), bar, or inches of mercury (inHg). The choice of units often depends on the application and regional preferences.
3. Vacuum Ranges: Different vacuum gauges are designed for specific pressure ranges:
4. Gauge Types: Various gauge types are used for different vacuum ranges, such as:
5. Environmental Factors: Atmospheric pressure variations due to weather conditions and altitude can affect gauge readings and must be considered for precise measurements.
6. Calibration: Regular calibration of vacuum gauges is crucial to ensure accuracy, especially in critical applications like semiconductor manufacturing or scientific research.
Interpreting vacuum measurements accurately is essential for maintaining process control, ensuring product quality, and optimizing system performance in various industrial and scientific applications.
In the industry, the vacuum degree is also known as the ultimate relative pressure. The relationship can be expressed as:Vacuum Degree=Atmospheric Pressure−Absolute PressureVacuum Degree=Atmospheric Pressure−Absolute PressureFor standard conditions, the atmospheric pressure is generally taken as 101325 Pa (Pascal). The ultimate absolute pressure varies depending on the type of vacuum pump used:
Ultimate Relative Pressure
Relative pressure, often referred to as gauge pressure, measures the pressure inside a container relative to the atmospheric pressure. It indicates the actual system pressure that is lower than the atmospheric pressure. When the air inside the container is pumped out, the internal pressure drops below the external atmospheric pressure. Therefore, when expressing this pressure using relative or gauge pressure, a negative sign is used to indicate that the internal pressure is lower than the external pressure.
Ultimate Absolute Pressure
Ultimate Absolute Pressure refers to the pressure inside a container compared to a perfect vacuum, which has a pressure value of 0 Pa. Due to technical limitations, achieving a perfect vacuum (0 Pa) is impossible. Thus, the vacuum level achieved by a vacuum pump is always higher than the theoretical vacuum value. When expressing this value in terms of absolute pressure, there is no need for a negative sign. For instance, if a device’s vacuum level is marked as 0.098 MPa, it means the absolute pressure inside the container is 0.098 MPa above the perfect vacuum.
Pumping Capacity
Pumping Capacity is a critical factor that measures the pumping speed of a vacuum pump, typically expressed in units such as liters per second (L/s) or cubic meters per hour (m³/h). It accounts for the system’s leakage rate.
A vacuum pump with high pumping capacity can more easily achieve the desired vacuum level, while a pump with low capacity may be slow or unable to reach the desired vacuum level when pumping the same volume of a container. This is because it is impossible to completely prevent gas leakage from the pipeline or container, and a high pumping capacity compensates for the vacuum decrease due to leakage.
Therefore, selecting a vacuum pump with a higher pumping capacity is recommended when calculating the theoretical pumping capacity. The formula for calculating pumping capacity will be introduced below.
Example Calculation
For example, if the vacuum degree of a device is marked as 0.098 MPa, in reality, it is -0.098 MPa when expressed in gauge pressure. However, in absolute terms, it remains 0.098 MPa above the perfect vacuum.
The conversion methods between Pa, KPa, MPa, mbar, bar, mmH2O, Psi are shown in the following table:
Conversion Table for Commonly Used Pressure Units in Laboratories
Units | Pa | KPa | MPa | bar | mbar | mmH2O | mmHg | p.s.i |
Pa | 1 | 10-3 | 10-6 | 10-5 | 10-2 | 101.97×10-3 | 7.5×10-3 | 0.15×10-3 |
KPa | 103 | 1 | 10-3 | 10-2 | 10 | 101.97 | 7.5 | 0.15 |
MPa | 105 | 103 | 1 | 10 | 104 | 101.97×103 | 7.5×103 | 0.15×103 |
bar | 105 | 102 | 10-1 | 1 | 103 | 10.2×103 | 750.06 | 14.5 |
mbar | 102 | 10-1 | 10-4 | 10-3 | 1 | 10.2 | 0.75 | 14.5×10-3 |
mmH2O | 10-1 | 9.807×10-3 | 9.807×10-6 | 98.07×10-6 | 98.07×10-3 | 1 | 73.56×103 | 1.42×10-3 |
mmHg | 9.807×10-3 | 133.32×10-3 | 133.32×10-6 | 1.33×10-3 | 1.33 | 13.6 | 1 | 19.34×10-3 |
p.s.i | 133.32×10-3 | 6.89 | 6.89×10-3 | 68.95×10-3 | 68.95 | 703.07 | 51.71 | 1 |
Selecting the right vacuum pump is crucial for ensuring the efficiency and effectiveness of the process it supports. Here are the key considerations to keep in mind:
1. The required vacuum degree for process
The working pressure of the vacuum pump must meet the process requirements. The selected vacuum degree should be half to one order of magnitude higher than that of the vacuum equipment. For instance, if the required vacuum degree in absolute pressure is 100 Pa, the vacuum pump selected should have a vacuum degree of at least 50-10 Pa.
2. The required pumping capacity for the process
The pumping speed of the vacuum pump, which is its ability to discharge gas, liquid, and solid substances under its working pressure, is typically expressed in units such as m³/h, L/s, and m³/min. The specific calculation for selecting a vacuum pump can be based on the following formula:
Where:
3. Determining the composition of the object being pumped
Understanding the nature of the object being pumped is essential for selecting the appropriate vacuum pump:
By considering these factors, you can select a vacuum pump that meets the specific needs of your process, ensuring optimal performance and longevity.
Vacuum systems are essential in various industrial and scientific applications, requiring different vacuum levels to function effectively. To achieve these varying vacuum levels, a combination of vacuum pumps operating in different pressure ranges is often necessary. This article explores the configuration and pumping speed considerations for vacuum systems, focusing on the use of high, medium, and low vacuum pumps.
Direct-Venting Vacuum Pump
The simplest vacuum system configuration is a direct-venting vacuum pump, which discharges directly to the atmosphere. This setup is suitable for low vacuum applications but is inadequate for achieving high vacuum levels.
Multi-Stage Vacuum Units
For high and medium vacuum systems, multi-stage units are typically required:
Creating an effective high vacuum system using just one high vacuum pump and one low vacuum pump is challenging due to several factors:
High vacuum pumps have limitations on the pressure they can handle at the front stage. If the pre-stage pressure exceeds a certain threshold, the high vacuum pump cannot function properly. This pressure limitation can lead to a decrease in pumping speed, disrupting the flow continuity and causing the vacuum unit to malfunction.
To address the flow continuity issue, a medium vacuum pump can be introduced between the high and low vacuum pumps. This intermediate pump ensures that all pumps operate within their optimal pressure ranges. Roots pumps, also known as Roots booster pumps, are ideal for this purpose due to their ability to operate effectively in the medium vacuum range (several Pa to several hundred Pa).
Pre-Pumping Time
The pre-pumping time is crucial, especially for vacuum units with periodic pumping requirements. Traditional high vacuum pumps have an initial working pressure in the range of several Pa, necessitating pre-pumping by the pre-stage pump to this pressure before the main pump can start. This process can be time-consuming, as the pumping speed decreases with pressure.
Molecular Booster Pumps
Molecular booster pumps offer a solution by combining medium and high vacuum performance. They have a high compression ratio and strong pumping capacity in the medium vacuum range, making them suitable for replacing traditional multi-stage units. These pumps can operate efficiently in the range of 100-50 Pa, significantly reducing the pre-pumping burden and enhancing overall system efficiency.
Simplifying High Vacuum Units
Molecular booster pumps can simplify high vacuum units by eliminating the need for Roots pumps. For large-scale high vacuum applications, strengthening the pre-pumping capability of the pre-stage pump can further reduce pumping time. The pre-stage pump can also serve multiple devices, streamlining the vacuum unit configuration.
Medium Vacuum Applications
In medium vacuum applications requiring pressures in the range of 10-1 Pa, a three-stage Roots pump unit is often necessary. However, molecular booster pumps can achieve full pumping speed at 10-1 Pa, making them a viable replacement for two-stage Roots pumps in a three-stage medium vacuum unit.
The configuration and pumping speed of vacuum systems are critical for achieving the desired vacuum levels efficiently. By understanding the limitations and capabilities of different vacuum pumps, such as high, medium, and low vacuum pumps, and incorporating advanced solutions like molecular booster pumps, it is possible to optimize vacuum system performance and simplify their configurations. This approach ensures that vacuum systems operate effectively across various applications, from industrial processes to scientific research.