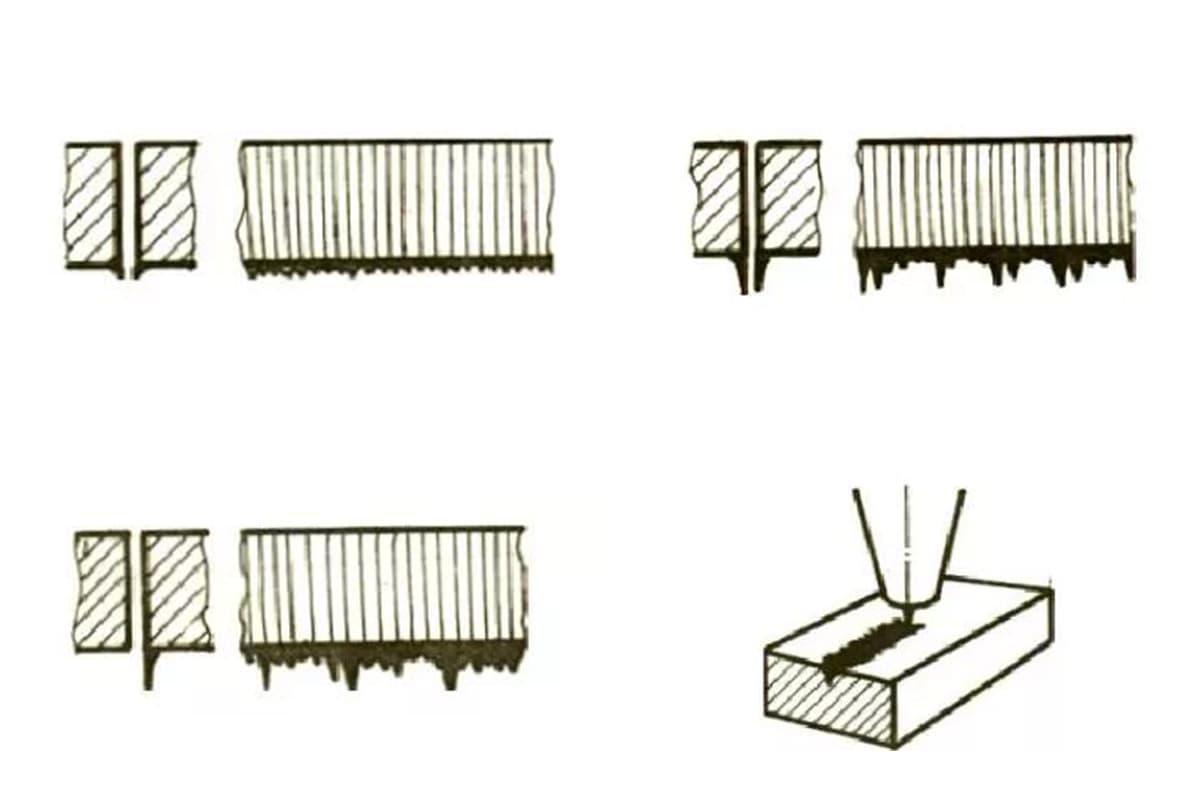
Ever wondered why laser-cut sheet metal sometimes falls short of perfection? This article addresses common laser cutting problems, like burrs on workpieces and incomplete cuts, and offers practical solutions. By understanding perforation techniques and adjusting parameters, you can enhance your cutting precision and efficiency. Whether you’re troubleshooting burrs on stainless steel or optimizing perforation methods, this guide equips you with the know-how to achieve flawless results. Dive in to transform your laser cutting process from problematic to perfect.
As a general rule, drilling a small hole in the sheet metal is necessary for any hot cutting process, with only a few exceptions where cutting can start from the edge of the sheet metal.
In the past, a hole was punched using a punch mold in a laser stamping machine before the laser cutting process began.
There are two fundamental methods for laser cutting without the use of a stamping device:
During continuous laser irradiation, a localized melt pool forms in the material’s center. This molten material is rapidly ejected by the high-pressure oxygen assist gas accompanying the laser beam, resulting in the formation of a through-hole.
The dimensions of the perforation are primarily influenced by the plate thickness, laser power, and assist gas parameters. Typically, the average diameter of the blast perforation is approximately 50-60% of the plate thickness. As plate thickness increases, perforations tend to become larger and may deviate from a circular shape due to heat affected zone expansion and gravity effects on the molten material.
This method is generally not recommended for components requiring high precision or tight tolerances. It is most suitable for rapid hole creation in non-critical areas or scrap material. The process can be optimized for specific applications by adjusting laser parameters and gas flow.
It’s important to note that the oxygen pressure used during the perforation process is often similar to that used in cutting operations. This high pressure, while effective for material removal, can lead to excessive splashing and potential surface contamination around the perforation site. For applications requiring cleaner perforations, alternative assist gases like nitrogen or argon may be considered, albeit at the cost of reduced cutting speed.
A high-peak-power pulsed laser is employed to rapidly melt or vaporize localized material. Inert gases such as nitrogen or clean compressed air are utilized as auxiliary gases to mitigate hole expansion caused by exothermic oxidation. The gas pressure is maintained lower than that used in oxygen-assisted cutting. Each laser pulse generates microdroplets that are ejected, gradually penetrating the material. Consequently, perforating thick plates may require several seconds.
Upon completion of perforation, the auxiliary gas is swiftly switched to oxygen for cutting initiation. This technique results in a smaller perforation diameter and superior hole quality compared to conventional blast perforation methods. To achieve this, the laser system must not only possess higher output power but also exhibit precise spatial and temporal beam characteristics. Standard flow CO2 lasers typically fall short of these stringent requirements.
Furthermore, pulse perforation necessitates a sophisticated gas control system capable of precisely regulating gas type, pressure, and perforation duration. To ensure high-quality cuts during pulse perforation, the transition from pulsed perforation to continuous cutting must be meticulously managed.
Theoretically, cutting parameters such as focal length, nozzle standoff distance, and gas pressure can be adjusted during the acceleration period. However, in industrial applications, modulating the laser’s average power proves more practical and efficient. This can be accomplished by altering the pulse width, frequency, or a combination of both. Extensive research has demonstrated that the latter approach, simultaneously adjusting both pulse width and frequency, yields optimal results in terms of cut quality and process stability.
When cutting small holes with high-power laser systems, deformation and quality issues can arise due to the energy concentration in a confined area. Traditional pulse perforation (soft puncture) techniques, while effective for less powerful systems, may lead to charring and hole distortion in high-power applications.
The primary cause of this phenomenon is the intense localization of laser energy during pulse perforation. This concentrated heat input can result in excessive material melting, vaporization, and thermal stress in the surrounding non-processing area. Consequently, the hole geometry becomes compromised, and the overall processing quality deteriorates.
To mitigate these issues in high-power laser cutting systems, it is recommended to transition from pulse perforation to blasting perforation (also known as single-pulse piercing or ordinary puncture). This method utilizes a single, high-energy pulse to rapidly create the initial hole, reducing the heat-affected zone and minimizing material distortion.
Key advantages of blasting perforation for small hole cutting with high-power lasers include:
Conversely, for lower-power laser cutting machines, pulse perforation remains the preferred method for small hole cutting. This technique offers several benefits in less powerful systems:
When cutting low carbon steel with CO2 laser technology, burr formation can be a significant issue. Understanding the root causes and implementing appropriate solutions is crucial for achieving clean, precise cuts. Here are the primary factors contributing to burr formation and their respective remedies:
When cutting low-carbon steel, stainless steel, or aluminum-zinc plates with a laser cutter, burr formation is a common challenge that requires careful consideration of multiple factors. The root causes of burrs can vary depending on the material properties and cutting parameters.
For low-carbon steel, initial investigation should focus on key factors influencing burr formation, such as laser power, cutting speed, focal point position, and assist gas pressure. However, simply increasing the cutting speed is not always an effective solution, as it may compromise the laser’s ability to fully penetrate the material, especially when processing thicker plates or highly reflective materials like aluminum-zinc alloys.
In the case of aluminum-zinc plates, which are known for their high thermal conductivity and reflectivity, additional considerations are necessary. The laser’s interaction with these materials can be more complex, often requiring a fine balance between power, speed, and focal point adjustment to achieve clean cuts with minimal burr.
To optimize cutting performance and reduce burr formation, consider the following factors:
After comprehensive analysis, the following factors have been identified as the primary contributors to unstable laser cutting processes:
Additional factors that may contribute to unstable processing include:
Abnormal spark patterns during the laser cutting of low carbon steel can significantly impact the quality of cut edges and overall part precision. If other cutting parameters are within normal ranges, consider the following potential causes and solutions:
The Working Principle of Laser Beam Cutting:
During the laser cutting process, the focused laser beam creates a localized melt pool on the material surface. As the beam continues to irradiate, it forms a depression at the center. High-pressure assist gas, coaxial with the laser beam, rapidly expels the molten material, creating a keyhole. This keyhole serves as the initial penetration point for contour cutting, analogous to a pilot hole in conventional machining.
The laser beam typically travels perpendicular to the tangent of the cut contour. Consequently, as the beam transitions from initial penetration to contour cutting, there’s a significant change in the cutting vector. Specifically, the vector rotates approximately 90°, aligning the cutting direction with the contour tangent.
This rapid vectorial shift can lead to surface quality issues at the transition point, potentially resulting in increased roughness or kerf width variations.
In standard operations where surface finish requirements are not stringent, automated CNC software generally determines puncture points. However, for applications demanding high surface quality or tight tolerances, manual intervention becomes crucial.
Manual adjustment of the puncture point involves strategically repositioning the initial penetration location. This optimization aims to minimize the impact of the vector change on the cut quality. Factors to consider include:
By carefully selecting the puncture point, engineers can significantly improve the overall cut quality, reducing post-processing requirements and enhancing part precision. Advanced techniques such as ramping or dimpling may also be employed to further optimize the penetration process.
It’s important to note that while manual puncture point selection can yield superior results, it requires expertise and may increase programming time. Therefore, a cost-benefit analysis should be conducted to determine when this level of optimization is warranted.