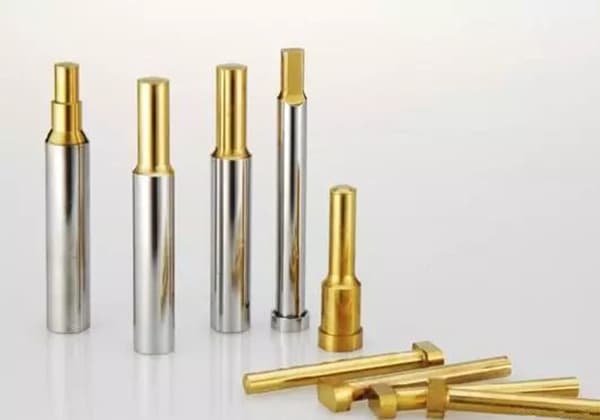
What materials make stamping dies both robust and precise? Stamping dies require high durability and precision, and materials like steel, carbide, and various alloys play critical roles. This article explores the pros and cons of carbon tool steel, high-speed steel, and innovative materials like steel-bonded carbide. Learn how each material impacts the performance and longevity of stamping dies, and understand which is best suited for your specific manufacturing needs. Dive into the essentials of stamping die materials and optimize your production process.
The materials used in the production of stamping dies include steel, cemented carbide, steel-bonded cemented carbide, zinc-based alloys, low-melting point alloys, aluminum bronze, and polymer materials.
At present, the primary material used for manufacturing stamping dies is steel. The commonly used materials for the working parts of dies include carbon tool steel, low alloy tool steel, high-carbon high chromium or medium chromium tool steel, medium carbon alloy steel, high-speed steel, base steel, hard alloy, and steel-bonded hard alloy.
Carbon tool steels, such as T8A and T10A, are extensively utilized in mold manufacturing due to their excellent machinability and cost-effectiveness. These steels typically contain 0.7% to 1.3% carbon, providing a good balance of hardness and toughness after heat treatment. Their favorable processing characteristics allow for efficient machining, grinding, and polishing, making them suitable for a wide range of mold applications.
However, carbon tool steels have limitations that must be considered in mold design and production:
Despite these drawbacks, carbon tool steels remain a viable option for many mold applications, particularly for short production runs, low-temperature molding processes, or when frequent mold replacement is economically feasible. To mitigate some limitations, surface treatments such as nitriding or hard chrome plating can be applied to enhance wear resistance and surface hardness.
Low alloy tool steel is an advanced variant of carbon tool steel, engineered with carefully selected alloying elements to enhance its mechanical and metallurgical properties. This class of steel typically contains 1-5% of total alloying elements, which significantly improve its performance characteristics compared to plain carbon tool steels. The addition of elements such as chromium, tungsten, manganese, vanadium, nickel, and molybdenum in precise proportions results in a material that exhibits superior hardenability, wear resistance, and dimensional stability during heat treatment.
In contrast to carbon tool steel, low alloy tool steel offers several key advantages:
Several grades of low-alloy steels are commonly employed in mold manufacturing, each tailored for specific applications:
When selecting a low alloy tool steel for mold manufacturing, factors such as mold size, complexity, production volume, and operating conditions must be carefully considered to optimize performance and longevity.
High-carbon, high-chromium tool steels, such as Cr12, Cr12MoV, and Cr12Mo1V1 (AISI D2), are widely utilized in the tooling industry due to their exceptional properties. These materials exhibit excellent hardenability, superior wear resistance, and minimal dimensional changes after heat treatment. Their load-bearing capacity is second only to high-speed steels, making them ideal for high-stress applications in metal forming and cutting operations.
The superior wear resistance of these steels stems from their high carbon content (typically 1.4-2.2%) and significant chromium levels (11-13%), which form hard carbides during heat treatment. These carbides, primarily chromium carbides, contribute to the steel’s outstanding abrasion resistance and edge retention properties.
However, a notable challenge with these steels is their tendency for carbide segregation during solidification and primary processing. This segregation can lead to anisotropic mechanical properties and reduced overall performance. To mitigate this issue, manufacturers employ a series of thermomechanical treatments, including repeated upsetting and drawing operations. This process, involving axial upsetting and radial drawing, helps to break up carbide networks, refine the microstructure, and achieve a more uniform carbide distribution throughout the material.
The optimization of carbide distribution through these processes significantly enhances the steel’s performance, improving its toughness, machinability, and overall consistency. This is particularly crucial for precision tooling applications where dimensional stability and uniform wear characteristics are paramount.
When selecting and processing these tool steels, it’s essential to consider the specific application requirements, heat treatment protocols, and the potential need for advanced surface treatments like nitriding or PVD coatings to further enhance their performance in demanding environments.
High-carbon medium-chromium tool steels, such as Cr4W2MoV, Cr6WV, and Cr5MoV, are widely utilized in mold manufacturing due to their superior properties. These alloys typically contain 0.5-1.5% carbon and 4-6% chromium, striking an optimal balance between hardness and toughness. Their composition results in a microstructure characterized by finely dispersed carbides within a tempered martensite matrix.
Key advantages of these steels include:
These properties make high-carbon medium-chromium tool steels particularly suitable for injection molds, die-casting dies, and other tooling applications requiring a combination of wear resistance, toughness, and dimensional stability. When properly heat-treated, these steels can achieve hardness levels of 58-62 HRC while maintaining adequate toughness for most mold applications.
High-speed steel (HSS) stands out as the premier choice among die steels, offering unparalleled hardness, wear resistance, and compressive strength. Its exceptional load-bearing capacity makes it ideal for high-stress applications in tooling and mold making. The most commonly utilized HSS grades in mold manufacturing are:
To optimize the microstructure and mechanical properties, high-speed steel requires careful heat treatment and often benefits from forging processes. Forging improves carbide distribution, refines grain structure, and enhances overall performance. This step is crucial in achieving the material’s full potential in terms of wear resistance, toughness, and dimensional stability in mold applications.
The selection of the appropriate HSS grade depends on specific mold requirements, production volume, and cost considerations. While HSS offers superior performance, it’s important to balance its higher cost against the expected tool life and productivity improvements in mold design and manufacturing processes.
Base steels represent an advanced class of tool steels engineered by carefully modifying the composition of high-speed steels (HSS) through precise alloying and carbon content adjustment. This metallurgical optimization enhances their overall performance characteristics. These steels retain the core attributes of HSS while offering improved wear resistance, hardness, fatigue strength, and toughness.
The unique combination of properties in base steels makes them particularly suitable for cold working die applications. They offer a balanced profile of high strength and toughness, crucial for withstanding the cyclic stresses and impacts encountered in metal forming processes. Notably, base steels provide a cost-effective alternative to traditional high-speed steels, offering comparable performance at a lower material cost.
Several grades of base steels have gained prominence in die manufacturing:
These base steel grades offer die manufacturers a range of options to meet specific application requirements, balancing factors such as wear resistance, toughness, and cost-effectiveness in cold working operations.
Cemented carbides, particularly tungsten carbide-cobalt (WC-Co) composites, offer superior hardness and wear resistance compared to conventional die steels. These properties make them invaluable in high-wear applications within the molding industry. However, their relatively lower bending strength and toughness necessitate careful consideration in die design and application.
The performance of cemented carbides can be tailored by adjusting the cobalt content:
Recent developments in cemented carbide technology include:
When selecting cemented carbides for molding applications, consider:
While cemented carbides offer exceptional wear performance, their implementation often requires specialized design considerations, such as stress-relieving geometries and appropriate pre-stressing techniques, to mitigate their inherent brittleness and optimize their longevity in demanding molding environments.
Steel-bonded cemented carbide is an advanced composite material produced through sophisticated powder metallurgy techniques. This innovative material utilizes iron powder as the primary binder, enhanced with carefully selected alloy elements such as chromium, molybdenum, tungsten, and vanadium. The hard phase consists of titanium carbide (TiC) or tungsten carbide (WC), which imparts exceptional wear resistance and hardness to the composite.
The steel matrix in this material offers a unique combination of properties that addresses the limitations of traditional cemented carbides. By leveraging the ductility and toughness of steel, steel-bonded cemented carbides overcome the brittleness and processing challenges associated with conventional cemented carbides. This enables a wide range of secondary processing options, including cutting, welding, forging, and heat treatment, significantly enhancing the material’s versatility in manufacturing applications.
While the hardness and wear resistance of steel-bonded cemented carbides are slightly lower than those of traditional cemented carbides, they still substantially outperform conventional steels in these aspects. The material’s microstructure, characterized by a high concentration of uniformly dispersed carbides within the steel matrix, contributes to its superior mechanical properties. After optimized heat treatment processes, including quenching and tempering, the hardness of steel-bonded cemented carbides can reach an impressive 68 to 73 HRC (Rockwell C scale), making them suitable for demanding wear-resistant applications.
The unique combination of hardness, wear resistance, and processability makes steel-bonded cemented carbides particularly valuable in industries such as mining, oil and gas, and heavy machinery, where components are subjected to severe abrasive and erosive environments while requiring complex geometries or post-fabrication modifications.
In stamping dies, soft materials refer to steels with a hardness of approximately HRC 35. Common examples include 45# steel, A3, and Q235. These materials possess relatively low hardness and are valued for their good impact resistance and machinability.
Key characteristics of soft materials in press tools:
Hard materials in stamping dies are high-strength steels with a hardness range of HRC 58 to 62 or higher after heat treatment. Common examples include Cr12, Cr12Mo1V1, Cr12MoV, SKD-11, SKD-51, and W6Mo5Cr4V2 (tungsten steel).
Key characteristics of hard materials in press tools:
The selection between soft and hard materials in press tool design depends on the specific component function, required wear resistance, and overall tool performance objectives. Optimal material selection and heat treatment processes are crucial for achieving the desired balance between hardness, toughness, and wear resistance in stamping die components.