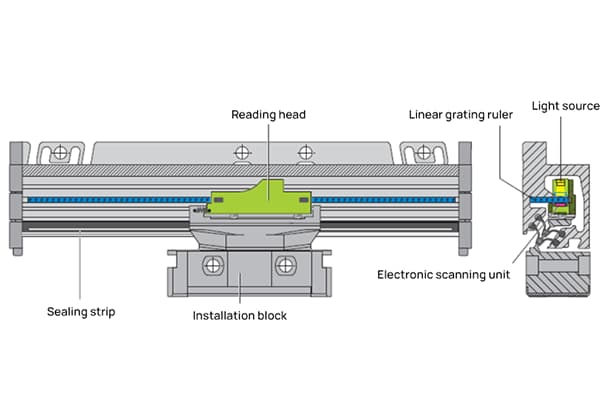
What causes steel ingots to crack during forging? This crucial question impacts industries reliant on robust metal components. This article delves into the factors behind cracking in 1Cr17Ni2 steel ingots, analyzing heating processes, chemical compositions, and structural integrity. Readers will learn essential prevention techniques, ensuring higher quality in future steel production and minimizing costly defects.
Two 4.6t steel ingots made of 1Cr17Ni2 developed serious transverse cracks during the forging process and one of them also had serious longitudinal cracks, which had a significant impact on the batch products. To avoid similar problems in future production, an analysis was conducted to determine the causes of the ingot cracking.
The ingot heating process involved keeping the ingot at 500℃ for 2 hours, then increasing the temperature to 850℃ at a rate of 100℃ per hour and maintaining that temperature for 2 hours. The temperature was then raised to 1180℃ and kept for 6 hours before the ingot was removed from the furnace for forging.
However, severe cracking occurred during the first fire compaction.
The surface of the ingot has numerous transverse cracks with wide openings. The longitudinal crack runs along the length of the ingot and is situated in the center of the billet. The crack opening is narrow and both ends of the ingot, the head and tail, are broken, as depicted in Figures 1 to 4.
The original fracture has oxidized and appears grayish-black, a morphology caused by the high temperature typical of fractures.
The cross-sectional test piece at the crack of the ingot underwent a hot acid leaching test. The results are displayed in Table 1.
Table 1 hot acid leaching test of cross-section test piece
General porosity / grade | Center porosity / grade | Ingot segregation / grade | Defect morphology |
1.5 | 2.5 | 3.5 | There are many cracks, the longest is about 6cm |
The cross-sectional test piece of the steel ingot is essentially square shaped, with an open crack located in the center of one side. The crack has a depth of approximately 6mm, which corresponds to the vertical depth of the macro longitudinal crack in the steel ingot.
The edge of the test piece exhibits a columnar crystal pattern and several small cracks, with a maximum length of around 10mm, as depicted in Figures 5 to 7.
The test results indicate that the ingot has severe shape segregation after undergoing forging (only pressing Square), making it unqualified. The small cracks observed are related to the height of the columnar crystals in the cast ingot.
The artificial fracture is a typical shell fracture, as shown in Fig. 8.
The test results reveal that the shell fracture is an abnormal fracture, and its formation requires further analysis.
Samples were taken from the surface of the steel ingot and from a location R/2 for chemical composition analysis. The results are presented in Table 2. The chemical composition was found to meet the technical requirements for 1Cr17Ni2 steel.
Table 2 chemical composition of 1Cr17Ni2 steel (mass fraction) (%)
Element | C | Mn | S | P | Si | Cr | Ni | Al |
Surface | 0.15 | 0.52 | 0.012 | 0.013 | 0.48 | 16.7 | 1.74 | 0.018 |
R / 2 | 0.15 | 0.53 | 0.012 | 0.013 | 0.49 | 16.8 | 1.77 | 0.018 |
A high magnification sample was taken from the test piece for the detection of non-metallic inclusions, and it was evaluated according to the microscopic inspection method for determining non-metallic inclusion content in steel as specified by the GB/T10561-2005 standard rating chart. The results can be seen in Table 3.
Table 3 test results of non-metallic inclusions (grade)
Position | Class A | Class B | Class C | Type D | Class Ds |
edge | 0.5 | 1.0 | 0.5 | 0.5 | 0.5 |
R / 2 | 1.0 | 1.5 | 0.5 | 0.5 | 0.5 |
core | 1.0 | 1.0 | 0.5 | 0.5 | 0.5 |
The ingot was found to be of qualified purity, but it contained many class B alumina inclusions.
The metallographic structure and grain size of samples taken from different positions were tested, and the results are displayed in Table 4.
Table 4 metallographic structure and grain size test
Position | Grain size / grade | Metallographic structure |
Edge | 5.0 | Low carbon ferrite + ferrite + intergranular carbide + lamellar structure |
R / 2 | 3.5 | Low carbon ferrite + ferrite + intergranular carbide + lamellar structure |
Core | 3.5 | Low carbon ferrite + ferrite + intergranular carbide + lamellar structure |
Columnar crystal region | 3.5 | Low carbon ferrite + ferrite + intergranular carbide + lamellar structure (structure distribution retains columnar crystal morphology) |
The test results indicate that the microstructure consists of low-carbon martensite, ferrite, intergranular carbides, and a lamellar structure. The carbides are evenly distributed along the grain boundaries and precipitated along the original columnar crystals, resulting in increased brittleness and decreased mechanical properties of the steel. The metallographic structure of each part is depicted in Figures 9 to 14.
The micro-morphology of the low magnification small cracks is characterized by varying widths, an intermittent appearance, fuzzy boundaries, and discontinuous tips. In addition, there are very fine cracks located near the small cracks that are arranged in an intermittent linear or island shape.
After being treated with a hydrochloric acid aqueous solution of high iron chloride, the microstructure of the small cracks and microcracks is primarily found along ferrite with a columnar crystal distribution. There is no significant change in the microstructure near the cracks, as demonstrated in Figures 15 to 18.
The results indicate that the small cracks and microcracks in the forgings are closely related to the carbides distributed along the columnar crystals in the cast state.
The macroscopic shell-like fracture is characterized by cleavage feathers and tear ridge lines that form between parallel cleavage when viewed under a scanning electron microscope. The cast free crystal surface and locally visible second-phase particles and inclusions can be seen, as depicted in Figures 19 to 22.
The source of the micro cleavage cracks is located on the free crystal surface at the grain boundary. Energy spectrum analysis revealed that it mainly contains elements such as C, Al, Si, Cr, Ni, among which Al, Si, Cr, and other elements have a higher composition than the average level, while the Ni element has a lower composition. The composition of the cleavage micro region is similar to the macro chemical composition.
The results indicate that the shell-like fracture is caused by micro segregation of aluminum in the steel.
The results of the chemical composition test indicate that the ingot material meets the technical specifications for 1Cr17Ni2 steel. However, the uniformity of the ingot’s structure is poor and the ingot segregation has been rated as a grade 3.5, which is considered unqualified.
The segregation of the ingot is due to the accumulation of impurities and component segregation at the junction between the columnar crystal region and the central equiaxed crystal region. Additionally, there are numerous small cracks in the low magnification columnar crystal region, with a micro crack morphology that resembles the carbide morphology of as-cast columnar crystals.
The post-forging structure of the ingot is composed of low-carbon martensite, ferrite, carbide, and a lamellar structure, with a grain size of 3.5-5.0. The structure in the columnar crystal region still retains its columnar shape, with a large number of continuously distributed carbides on the grain boundary, which contributes to the brittleness of the structure.
The shell-like fracture in the columnar crystal region of the ingot is an abnormal fracture, with a micro fracture that exhibits cleavage and tear ridges, indicating the ingot’s brittleness. The source of the micro cleavage crack is located on the free crystal surface of the grain boundary and is caused by the presence of Cr-containing carbides and a second phase containing Al.
When the aluminum content exceeds 0.09%, the shell-like fracture is more likely to occur in the columnar crystal region. During the aluminum deoxidation process, if the aluminum content is not strictly controlled, a significant amount of aluminum residue may result. Although the original aluminum content in the molten steel meets the standards, due to the low melting point of aluminum, the concentration of aluminum in the residual molten steel increases significantly, leading to the precipitation of a second phase containing aluminum in dendrite form, which is a type of micro segregation.
When the crystallization process is slow, the dendrite aluminum-containing second phase precipitates from the residual molten steel and is pushed to the grain boundary of the primary crystallization. If the matrix crystallization speed exceeds a critical speed, the second phase is trapped in the growing crystal and eventually increases the intergranular fracture sensitivity.
The heating process for the steel ingot involves heating to 500°C for 2 hours, then heating to 850°C at a rate of 100°C per hour for 2 hours, heating to 1180°C for 6 hours, and finally being removed from the furnace for forging.
1Cr17Ni2 is a martensitic-ferritic duplex stainless steel that exhibits brittleness at 475°C. It is advised to avoid extended heating in the temperature range of 400-525°C. When 1Cr17Ni2 steel is heated above 900°C, its grain growth tendency increases, causing an increase in brittleness and worsening forging conditions.
The results of the chemical composition analysis show that the ingot material conforms to the technical specifications for 1Cr17Ni2 steel. However, the uniformity of its microstructure is inadequate, and the ingot segregation is significant.
The post-forging structure of the steel ingot is poor, with the main cause being an improper heating process design that increases the risk of brittleness at 475°C. Furthermore, the slow cooling of the ingot during casting leads to the precipitation of an aluminum-containing phase in the columnar crystal region, which increases the sensitivity to intergranular fractures.
These two factors combine to cause cracking during forging.