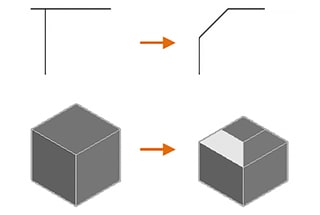
Have you ever wondered how sharp edges on metal parts are smoothed out? This process, known as chamfering, transforms dangerous, jagged corners into safer, angled surfaces. In this article, you’ll learn the different types of chamfering, their purposes, and the methods used, ensuring safer and higher-quality mechanical components.
Chamfering is a precision metal fabrication process that involves creating an angled or beveled surface on the edge or corner of a workpiece. This technique is widely used in manufacturing to modify the transition between two surfaces, typically at a 45-degree angle, though other angles can be employed based on specific design requirements.
In essence, chamfering entails removing material to transform sharp edges into angled planes or rounded profiles. The resulting chamfer can take various forms, including:
The chamfering process serves multiple purposes in metal fabrication:
Chamfers can be produced through various manufacturing methods, including machining, grinding, filing, or specialized chamfering tools, depending on the material, precision requirements, and production volume.
C Chamfering refers to the processing of a specified angled surface on the corner of a material. The term chamfering often refers to C-type chamfering.
In drawings, it is marked as ←C0.5 at the edge position, or “unspecified face C0.5”, etc.
Here, C0.5 refers to a 0.5mm sloping surface machined at 45° from the edge. Note that it does not refer to the length of the slope.
R Chamfering refers to the processing of the corner of a material into an arc shape. On the drawing, it is specified as “should do R chamfering”, etc.
“R chamfering” is sometimes also referred to as “R processing” or “Round processing”.
Line chamfering refers to the processing of a surface on the corner of a material that is invisible to the naked eye.
Line chamfering is generally considered to be about C0.2~0.3, but unlike C chamfering and R chamfering, there are no clear regulations on the shape and size of the chamfer.
In drawings, it is often marked as “unspecified corner doing line chamfering” or “each edge must be free of burrs”.
Enhancing Safety
Mechanical processing often results in sharp corners and burrs on material edges. These can pose significant safety risks, potentially causing lacerations if handled without proper protection. Chamfering effectively eliminates these hazards by creating a beveled edge, substantially reducing the risk of injury during handling and assembly processes.
Quality Improvement
The presence of sharp edges or burrs can lead to various quality issues. When components interact, these imperfections may cause surface scratches, compromising both aesthetics and functionality. Moreover, loose burrs can detach during operation, potentially causing contamination or mechanical failures in precision systems.
During cutting and stamping operations, the edges of workpieces often experience plastic deformation, resulting in edge warping or distortion. This can lead to poor fit tolerances or component damage during forced assembly. Chamfering mitigates these risks by creating a uniform, controlled edge profile, ensuring better part compatibility and reducing the likelihood of assembly-related defects.
Enhancing Assembly Performance
Chamfered edges significantly improve assembly efficiency and precision. By creating a tapered lead-in, chamfers act as a guide, facilitating smoother component mating and reducing the risk of misalignment during assembly.
In applications where cylindrical components are inserted into holes, even minor discrepancies between the inner diameter of the hole and the outer diameter of the component can impede smooth insertion, especially if there’s slight misalignment or angular deviation. Chamfering both the hole entrance and the component end creates a funnel-like effect, allowing for easier initial engagement and self-centering during insertion. This tolerance for misalignment within the chamfer range greatly enhances assembly speed and reduces the risk of component damage due to forced insertion.
Chamfering can be done in various ways such as milling, lathe turning, manual work, etc. Here, we introduce the chamfering processing method through milling.
Milling is a process that involves pressing a rotating cutter onto a workpiece fixed on a slide table.
By using a chamfer cutter designed according to the shape of the workpiece, chamfering can be easily achieved.
In the case of C chamfering, chamfering can also be accomplished by tilting the tool or workpiece and using a general flat-end mill.
Key points in processing include the following two points.
For R chamfering, please refer to the following.
Ideally, the amount of cutting in the Ad and Rd directions should be approximately the same.
Different cutting depths should be used for roughing and finishing.
Turning chamfering uses a lathe to create precise chamfers on cylindrical workpieces. This technique is particularly useful for parts that need accurate, symmetrical chamfers around their edges.
Milling chamfering uses milling machines to create chamfers on both flat and irregular surfaces.
Grinding chamfering is used for finishing edges with high precision and smoothness, often applied to hardened materials.
Laser chamfering uses a focused laser beam for precise chamfers with minimal thermal distortion.
Waterjet chamfering uses high-pressure water mixed with abrasive particles to cut chamfers without heat.
Plasma chamfering uses a plasma torch for cutting chamfers on conductive materials, ideal for thick and high-alloy metals.
Hand chamfering uses manual tools for small-scale or precision work where machine chamfering is impractical.
Automated chamfering is used in high-volume production environments, integrating chamfering into automated machining processes.
By employing these various chamfering techniques, machinists can select the most appropriate method based on the material, desired precision, and production volume, ensuring high-quality chamfers for diverse applications.
There are several types of chamfers in the components depicted in the blueprints, including edge chamfer, hole chamfer, shaft-end chamfer, and the removal of sharp edges and burrs.
1. Edge Chamfer:
Also known as external edge chamfer. For example, a cube has 12 external edges. If the blueprint indicates a chamfer of C0.5, then all 12 edges should be processed into a chamfer of 0.5*45°.
2. Hole Chamfer:
This includes circular holes and irregular holes. If the blueprint indicates a hole chamfer of C0.5, then all holes in the component should be processed to a chamfer of 0.5*45°. If only a specific part is required, it should be clearly marked.
3. Shaft-end Chamfer:
This refers to the chamfer at both ends of a shaft. For step shafts, if it needs to be specified in text, it must be labeled as a shaft shoulder chamfer. Assume a step shaft designer requires all shaft shoulders and both ends of the shaft to have a chamfer of 0.5*45°, it can be written as shaft end and shoulder chamfer C0.5.
Note: If only “shaft-end chamfer C0.5” is written, the absence of a shoulder chamfer does not constitute a returnable defect. If only “shaft shoulder chamfer C0.5” is written, the absence of an end chamfer does not constitute a returnable defect.
4. Chamfering of disc-shaped parts:
The chamfer of disc-shaped parts cannot be written as shaft end chamfer. It must be drawn and labeled on the diagram.
5. Chamfering of threaded holes and screw ends:
It is agreed to chamfer to the thread depth, and there is no need to explain it on the drawing. If there are special circumstances, they must be specifically stated.
6. Deburring:
This is also a way to describe chamfering, specifically used in the process of sheet metal parts. For example, it is not appropriate to talk about chamfering a 1mm thin plate. Now, it is stipulated that the chamfering process for plates under 3mm thick, which is used for smooth touch requirements, is all called deburring.
7. Used for filleting corners:
The process used for filleting corners needs to be written as R<… (Note: from a process perspective, please take as large a value for R as possible) or to create a clearance hole.
Note: Chamfering a C-angle is cheaper than chamfering an R-angle (for external contours).
The following statements are correct:
1. The drawings indicate an unspecified chamfer of C1, but nowhere on the drawings is a chamfer explicitly drawn or depicted, making the mention of an unspecified chamfer meaningless. (This point needs serious attention.)
2. The edges of holes and the straight edges of square holes in the parts are not considered text chamfers.
3. Depending on the actual conditions of the parts, the number of chamfers mentioned above sometimes exceeds 12. For instance, when a groove is cut into a plate, the two edges of the groove are additional external chamfers, and the original chamfer is divided into multiple external chamfers by the groove, while the chamfers at the bottom of the groove or recess do not count as external chamfers.
4. The chamfers at the bottom of the recess are not considered external chamfers.
5. Chamfering is only used for external chamfers.
6. If the drawings indicate a certain number of chamfers, there is no need to depict the shapes of those chamfered external edges in the drawings. This also applies to chamfers at the edges of holes or ends of shafts, and shoulder chamfers.
7. Sharp or obtuse angles should not be marked on the blueprint, as edges are typically right angles (90° should not be referred to as sharp angles).
8. Chamfers also include external edges with acute angles.
9. To ensure the unambiguity of the blueprint, an extra view is often drawn, even if no dimensions are marked on it.
A chamfer is a beveled edge that connects two surfaces at an angle, usually around 45 degrees. It’s used to remove sharp edges, making parts safer to handle and easier to assemble, while also enhancing their appearance. Chamfers are common in many industries to make parts fit together more easily, reduce stress, and improve the product’s look.
A countersink creates a conical hole in a material, allowing the head of a screw or bolt to sit flush with or below the surface. Common angles for countersinks are 82, 90, 100, or 120 degrees. Countersinks are essential for applications where fasteners need to be flush with the surface for both functional and aesthetic reasons.
Deburring removes small, rough edges left on a part after machining operations like drilling, milling, or cutting. It can be done manually or automatically. The main goal is to improve the part’s finish, remove sharp edges, and ensure it meets safety and functionality standards.
Knowing the differences between chamfering, countersinking, and deburring helps you choose the right technique and tools for manufacturing and assembly, ensuring high-quality, safe, and functional products.
Below are answers to some frequently asked questions:
Chamfer forms vary based on their angle, thread size, and flute type, each serving specific applications. Form A, with a thread size of 6-8 and a 5° angle, uses straight flutes and is ideal for short through holes. Form B, featuring a thread size of 3.5-6 and an 8° angle, also uses straight flutes with a spiral point, suitable for through holes in medium or long chipping materials. Form C, with a thread size of 2-3 and a 15° angle, employs straight or spiral flutes, perfect for blind or through holes in short chipping materials. Form D, with a thread size of 3.5-5 and an 8° angle, uses straight or spiral flutes for blind holes with thread exit or through holes. Lastly, Form E, with a thread size of 1.5-2 and a 23° angle, utilizes straight or spiral flutes, suitable for blind holes with short thread exit. These chamfer forms are crucial in machining for easing edges, improving safety, and facilitating assembly.
To perform chamfering using techniques like turning and milling, you need to follow specific procedures for each method.
For turning, internal chamfering involves using an internal diameter tool to machine the inner edge of a hole, positioning the tool to cut at the desired angle. External chamfering is done with an external tool to cut the outer edge of a workpiece, typically set to a 45-degree angle.
For milling, chamfer milling uses specialized tools such as chamfer mills, face mills, and end mills. Chamfer mills create various edge profiles by rotating the cutter at a slow speed and feeding it into the workpiece at a moderate rate. Face mills can chamfer edges of different sizes and shapes, while end mills are tilted to the desired angle as they pass through the workpiece. Using chamfer cutters or solid head replaceable chamfer tools ensures precise angles for both internal and external chamfering. Step or dwell machining techniques help manage chip fragmentation during milling.
Accurate chamfering requires proper machine setup, including zeroing the tool and using seated parallels or tilting the mill head. Operating at slow speeds and moderate feed rates prevents overheating and maintains control. Ensuring tool alignment and using appropriate cooling methods are also essential for maintaining tool integrity and achieving consistent results.
The best tools for chamfering include chamfer mills, hand-held chamfering tools, and external and internal deburring chamfer tools. Chamfer mills, especially carbide ones, are ideal for precision work on hard materials like tool steel, offering durability and cutting efficiency. Hand-held tools, such as pneumatic bevelling tools and carbide bits in routers, are preferred for manual operations and are suitable for creating precise chamfers on various materials, including aluminum. External and internal deburring chamfer tools, like those from ORX PLUS, are versatile and can be attached to power drills for easy and efficient operation, making them suitable for a wide range of materials. The choice of tool depends on the material being chamfered, required precision, durability, cost, and safety features.
Chamfers are crucial for safety and assembly in various industries. They eliminate sharp edges, significantly reducing the risk of injuries during handling or use of components, which is vital for consumer products like furniture and tools. Chamfers also prevent material fatigue by distributing stress more evenly, enhancing the durability and longevity of products. In assembly, chamfers facilitate the alignment and insertion of parts, ensuring a smooth and precise fitting, which is essential for mechanical and industrial applications. Additionally, chamfers provide clearance for interior radii, preventing interference issues during assembly. These benefits make chamfers an essential element in manufacturing and design processes.
A chamfer is a beveled edge that transitions between two faces of an object, typically at an angle, often used to remove sharp edges, facilitate assembly, and enhance aesthetics. A countersink is a specific type of chamfer applied to round holes, designed to allow the head of a fastener to sit flush with the surface, and it has more critical tolerances. Deburring, on the other hand, is the process of removing sharp edges or burrs resulting from machining operations, primarily to ensure safety and smooth operation, rather than being a deliberate design feature.
Choosing the right chamfer angle for your application involves understanding the specific requirements of your design and manufacturing process. Start by considering the standard angles used in your industry; for example, a 45-degree chamfer is common for general purposes due to its balance of strength and ease of machining. If you are working with pipes or tubing, a 37.5-degree angle might be more suitable, while aerospace applications often use angles like 100-110 degrees for rivets.
Additionally, think about the material you are working with and the functionality of the chamfer. For instance, a sharper angle might be necessary for aesthetic purposes or to reduce stress concentrations, while a broader angle could facilitate easier assembly or welding. Ensure that you use appropriate measuring tools like chamfer gauges to verify the angle and maintain precision. By carefully considering these factors, you can select the chamfer angle that best meets your application’s needs.