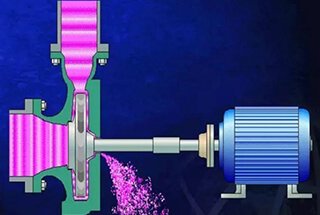
Imagine a world where nearly anything can be created layer by layer, from intricate medical implants to entire houses. This is the power of 3D printing. Our article explores the seven major types of 3D printing technologies, each with unique methods and applications. Discover how these technologies are transforming industries, and learn which methods are best for different materials and products. Whether you’re curious about bioprinting or the latest in metal fabrication, this guide will illuminate the fascinating world of additive manufacturing.
Many laypeople think that 3D printing is just about squeezing material out of a hot nozzle and stacking it into shapes, but 3D printing goes far beyond that! Today, we introduce seven types of 3D printing processes to help differentiate between various 3D printing techniques.
In fact, 3D printing, also known as additive manufacturing, is a general term that encompasses several distinctly different 3D printing processes. These technologies vary greatly, but the key processes are the same.
For instance, all 3D printing starts with a digital model because the technology is essentially digital. The part or product is initially designed using Computer-Aided Design (CAD) software or sourced from a digital parts library.
The design file is then broken down into slices or layers for 3D printing through specific build preparation software, generating path instructions for the 3D printer to follow.
Next, you will learn the differences between these technologies and the typical uses for each.
Why are there 7 types?
The types of additive manufacturing can be categorized by the products they produce or the types of materials they use. The International Standards Organization (ISO) has divided them into seven general types (although these seven categories of 3D printing can barely cover the increasing number of sub-types and hybrid technologies).
As the name suggests, material extrusion involves material being extruded through a nozzle.
Typically, this material is a plastic filament that is melted and extruded through a heated nozzle. The printer places the material on the build platform along the process path obtained through software. The filament then cools and solidifies into a solid object. This is the most common form of 3D printing.
It may sound simple at first glance, but considering the extruded materials, including plastic, metal, concrete, bio-gels, and various foods, it is actually a very broad category. The price of this type of 3D printer ranges from $100 to seven figures.
The FDM 3D printer market is worth billions of dollars, with thousands of machines ranging from basic models to complex manufacturer models. FDM machines are referred to as Fused Filament Fabrication (FFF), which is entirely the same technology.
Like all 3D printing technologies, FDM starts with a digital model and then converts it into a path that the 3D printer can follow. With FDM, a filament (or multiple filaments at once) from a line coil is loaded into the 3D printer and then fed into the printer nozzle in the extrusion head.
The printer nozzle or multiple nozzles are heated to the required temperature to soften the filament, allowing the continuous layers to bond together to form a solid part.
As the printer moves the extrusion head along the specified coordinates in the XY plane, it continues to lay the first layer. The extrusion head then rises to the next height (Z plane) and repeats the process of printing cross-sections, building layer by layer until the object is fully formed.
Depending on the geometry of the object, support structures may sometimes need to be added to support the model during printing, for instance, if the model has steep overhangs. These supports are removed after printing. Some support structure materials can dissolve in water or another solution.
3D bioprinting, or bio 3D printing, is an additive manufacturing process where organic or biological materials (such as living cells and nutrients) are combined to create natural three-dimensional structures similar to tissues.
In other words, bioprinting is a form of 3D printing that can produce anything from skeletal tissues and vessels to living tissues. It is used for various medical research and applications, including tissue engineering, drug testing and development, and innovative regenerative medical therapies. The actual definition of 3D bioprinting is still evolving.
Essentially, 3D bioprinting works similarly to FDM 3D printing and belongs to the material extrusion series (although extrusion is not the only bioprinting method).
3D bioprinting uses material (bio-ink) ejected from needles to create printed layers. These materials, known as bio-inks, primarily consist of living matter, such as cells in carrier materials — like collagen, gelatin, hyaluronic acid, silk, alginate, or nanocellulose, acting as molecular scaffolds for structural growth and nutrients, providing support.
Architectural 3D printing is a rapidly advancing field in material extrusion. This technology involves using gigantic 3D printers, often several meters tall, to extrude construction materials like concrete from a nozzle.
These machines typically appear in gantry or robotic arm systems. Today, 3D architectural printing technology is used in housing, architectural features, and various construction projects from wells to walls. Researchers suggest it has the potential to significantly transform the entire construction industry by reducing labor demand and minimizing construction waste.
Dozens of 3D printed houses exist in the United States and Europe, and research is being conducted into 3D construction technology that would use materials found on the moon and Mars to build habitats for future explorers. Replacing concrete printing with local soil as a more sustainable construction method is also gaining attention.
Vat Polymerization (also known as Resin 3D Printing) is a series of 3D printing processes that selectively cures (or hardens) photosensitive polymer resin in a vat using a light source. In other words, light is precisely directed to specific points or areas of the liquid plastic to harden it.
After the first layer is cured, the build platform moves up or down slightly (depending on the printer), usually between 0.01 and 0.05 millimeters, and the next layer is cured and connected to the previous one.
This process is repeated layer by layer until a 3D part is formed. After the 3D printing process is completed, the object is cleaned to remove any remaining liquid resin and post-cured (in sunlight or a UV chamber) to enhance the part’s mechanical properties.
The three most common forms of vat polymerization are Stereolithography (SLA), Digital Light Processing (DLP), and Liquid Crystal Display (LCD), also known as Masked Stereolithography (MSLA). The fundamental difference between these types of 3D printing technologies lies in the light source and how it is used to cure the resin.
Several 3D printer manufacturers, especially those producing professional-grade 3D printers, have developed unique and patented variations of photopolymerization, so you may see different technology names on the market.
Industrial 3D printer manufacturer Carbon uses a vat polymerization technology called Digital Light Synthesis (DLS), Stratasys’ Origin calls its technology Programmable Photopolymerization (P3), Formlabs offers its technology called Low Force Stereolithography (LFS), and Azul 3D is the first to commercialize vat polymerization on a large scale in a form called High Area Rapid Printing (HARP).
Other technologies include Lithography-based Metal Manufacturing (LMM), Projection Micro Stereolithography (PμSL), and Digital Composite Manufacturing (DCM), a filled photopolymer technology that introduces functional additives (like metal and ceramic fibers) into the liquid resin.
Stereolithography, or SLA, is the world’s first 3D printing technology. Invented in 1986 by Chuck Hull, who patented the technology and founded 3D Systems to commercialize it, SLA is now available to enthusiasts and professionals alike from numerous 3D printer manufacturers.
The process involves directing a laser beam onto a vat of resin, selectively solidifying cross sections of the object within the print area in a layer-by-layer build. Most SLA printers use a solid-state laser to solidify the parts.
A drawback of this vat polymerization is that, compared to our next method (DLP), point lasers may take longer time to trace the object’s cross-section, which flashes light to instantly harden the entire layer. However, lasers can produce stronger light, which is needed for some engineering-grade resins.
(1) Micro-Stereolithography (μSLA)
Micro-Stereolithography technology can print miniature parts with a resolution between 2 micrometers (μm) and 50 μm. For reference, the average width of a human hair is 75 μm. It’s one of the “micro 3D printing” technologies.
μSLA involves exposing photosensitive material (liquid resin) to an ultraviolet laser. What differentiates it is the specialized resin, the complexity of the laser, and the addition of lenses that produce incredibly small light points.
(2) Two-Photon Polymerization (TPP)
Another micro 3D printing technology, TPP (also known as 2PP), can be categorized under SLA because it also uses a laser and photosensitive resin. It can print parts smaller than μSLA, as small as 0.1 μm. TPP uses a pulsed femtosecond laser focused to a narrow point within a large vat of special resin.
That point is then used to solidify single 3D pixels, or voxels, within the resin. These tiny voxels, ranging from nano to micro size, are solidified layer by layer along a predefined path. TPP is currently used in research, medical applications, and the manufacture of micro parts such as microelectrodes and optical sensors.
DLP 3D printing uses a digital light projector (instead of a laser) to flash the image of each layer (or multiple exposures for larger parts) onto a layer or vat of resin. DLP (more common than SLA) is used to produce larger parts or larger volumes of parts in a single batch, as each layer exposure takes the same amount of time regardless of the number of parts in the build, making it more efficient than the point laser method in SLA.
The image of each layer is made up of square pixels, resulting in a layer made up of small rectangular blocks called voxels. Light is projected onto the resin using a light-emitting diode (LED) screen or a UV light source (lamp) and is projected onto the build surface through a digital micromirror device (DMD).
Modern DLP projectors typically have thousands of micro-sized LEDs as light sources. Their on-off states are individually controlled, increasing the XY resolution. Not all DLP 3D printers are the same, with significant differences in the power of the light source, the lens it passes through, the quality of the DMD, and many other components that make up a machine worth $300 compared to ones worth over $200,000.
Top-Down DLP
Some DLP 3D printers mount the light source at the top of the printer, shining down onto the vat of resin instead of upwards. These “top-down” machines flash an image of a layer from the top, solidifying one layer at a time, then returning the solidified layer back into the large vat.
Each time the build platform is lowered, a recoater mounted at the top of the large vat moves back and forth on the resin to even out the new layer. Manufacturers claim this method produces more stable part outputs for larger prints because the print process doesn’t fight against gravity.
There are limits to how much weight can be suspended vertically from the build plate during bottom-up printing. The vat of resin also supports the part during printing, reducing the need for support structures.
Projection Micro-Stereolithography (PμSL)
As a distinct type of vat polymerization itself, PμSL is classified under DLP as a subcategory. It’s another micro 3D printing technology. PμSL uses ultraviolet light from a projector to solidify layers of a special formula resin at the micron scale (2 μm resolution and as low as 5 μm layer height).
This additive manufacturing technology is evolving due to its low cost, accuracy, speed, and range of usable materials (including polymers, biomaterials, and ceramics). It has shown potential for applications from microfluidics and tissue engineering to micro-optics and biomedical microdevices.
Lithography-based Metal Manufacturing (LMM)
This distant relative of DLP is a method of 3D printing with light and resin that can create tiny metal parts for applications like surgical tools and micro-mechanical parts. In LMM, metal powder is evenly dispersed in a photosensitive resin, which is selectively polymerized via exposure to blue light from a projector.
After printing, the polymer component of the green part is removed, leaving a fully metallic debound part that is finalized in a sintering process in a furnace. Raw materials include stainless steel, titanium, tungsten, brass, copper, silver, and gold.
The Liquid Crystal Display (LCD), also known as Masked Stereolithography (MSLA), is very similar to the aforementioned DLP. The difference lies in the use of an LCD screen instead of a Digital Micromirror Device (DMD), which significantly affects the price of 3D printers.
Like DLP, the LCD light mask is digitally displayed and made up of square pixels. The size of the pixels on the LCD light mask determines the granularity of the print. As such, the XY accuracy is fixed and does not depend on the degree of zoom or scaling of the lens, as is the case with DLP.
Another difference between DLP printers and LCD technology is that the latter uses an array of hundreds of individual emitters rather than a single point light source like laser diodes or DLP lamps.
Like DLP, LCD can achieve faster print times than SLA under certain conditions. This is because the entire layer is exposed at once, rather than tracing the cross-sectional area with a laser point.
Due to the low unit cost of LCDs, this technology has become the preferred technology in the low-cost desktop resin printer field. However, this does not mean it isn’t used professionally. Some industrial 3D printer manufacturers are pushing the technological limits and achieving impressive results.
Powder bed fusion (PBF) is a 3D printing process in which a thermal energy source selectively melts powder particles (plastic, metal, or ceramic) within the build area to create solid objects layer by layer.
A PBF 3D printer disperses a thin layer of powdered material onto the print bed, typically using a blade, roller, or wiper. Energy from a laser fuses specific points on the powder layer, and then another layer of powder is deposited and fused to the previous layer. This process is repeated until the entire object is manufactured, with the final product encased and supported by unfused powder.
PBF can produce parts with high mechanical performance (including strength, wear resistance, and durability) for the end-use in consumer goods, machinery, and tools. The 3D printers in this submarket are becoming cheaper (starting at around $25,000), but it’s considered an industrial technology.
Selective Laser Sintering (SLS) uses a laser to manufacture objects from plastic powder. First, a box of polymer powder is heated to just below the polymer’s melting point. Then, a recoating blade or wiper deposits a very thin layer of powdered material (usually 0.1mm thick) onto the build platform.
The laser begins to scan the surface according to the pattern laid out in the digital model. The laser selectively sinters the powder and solidifies the object’s cross-section. When scanning the entire cross-section, the build platform moves down by one layer thickness. The recoating blade deposits a new layer of powder over the most recently scanned layer, and the laser sinters the object’s next cross-section onto the previously solidified cross-section.
These steps are repeated until all objects are manufactured. Unsintered powder remains in place to support the object, reducing or eliminating the need for support structures. After the part is removed from the powder bed and cleaned, no other necessary post-processing steps are required.
The part can be polished, coated, or colored. There are many differentiating factors between SLS 3D printers, not only their size but also the power and number of lasers, the size of the laser spot, the time and manner of heating the bed, and the distribution of powder. The most common material in SLS 3D printing is nylon (PA6, PA12), but TPU and other materials can also be used to print flexible parts.
μSLS belongs to the technology of SLS or the Laser Powder Bed Fusion (LPBF) mentioned below. It uses a laser to sinter powdered material, like SLS, but this material is typically metal rather than plastic, so it’s more akin to LPBF. It is another micro 3D printing technology that can create parts with micro resolution (below 5 μm).
In μSLS, a layer of metal nanoparticle ink is coated on the substrate, then dried to produce a uniform layer of nanoparticles. Next, a patterned laser from a digital micromirror array is used to heat the nanoparticles and sinter them into the desired pattern. This set of steps is repeated to build each layer of the 3D part in the μSLS system.
Among all 3D printing technologies, this one has the most aliases. The formal name of this metal 3D printing method is Laser Powder Bed Fusion (LPBF), but it is also widely known as Direct Metal Laser Sintering (DMLS) and Selective Laser Melting (SLM).
In the early development of this technology, machine manufacturers created their own names for the same process, and these names have been used ever since. Notably, the three terms above refer to the same process, even if some mechanical details differ.
As a subtype of powder bed fusion, LPBF uses a metal powder bed and one or more (up to 12) high-power lasers. LPBF 3D printers use lasers to selectively fuse metal powders together on a molecular basis layer by layer until the model is complete. LPBF is a highly precise 3D printing method, typically used to create complex metal parts for aerospace, medical, and industrial applications.
Like SLS, LPBF 3D printers start with a digital model divided into slices. The printer loads powder into the build chamber, then uses a scraper (like a windshield wiper) or roller to spread it into a thin layer on the build plate. The laser traces the layer onto the powder.
Then the build platform moves down, another layer of powder is applied and fused with the first layer until the entire object is built. The build chamber is enclosed, sealed, and often filled with an inert gas mixture, such as nitrogen or argon, to ensure that the metal does not oxidize during the melting process and helps clear debris from the melting process.
After printing, the part is removed from the powder bed, cleaned, and often undergoes secondary heat treatment to eliminate stress. The remaining powder is recycled and reused.
Differentiating factors of LPBF 3D printers include the type, strength, and number of lasers. Small compact LPBF printers may have a 30-watt laser, while industrial versions may have 12 1,000-watt lasers. LPBF machines use common engineering alloys, such as stainless steel, nickel superalloys, and titanium alloys. There are dozens of metals available for the LPBF process.
EBM, also known as Electron Beam Powder Bed Fusion (EB PBF), is a metal 3D printing method similar to LPBF but uses an electron beam instead of a fiber laser. This technology is used to manufacture parts, such as titanium orthopedic implants, turbine blades for jet engines, and copper coils.
The electron beam generates more energy and heat, which is needed for some metals and applications. Furthermore, EBM is not an inert gas environment but is carried out in a vacuum chamber to prevent beam scattering. The build chamber temperature can reach up to 1,000 °C, and even higher in some cases. Because the electron beam uses electromagnetic beam control, its movement speed is faster than the laser and can even be split to expose multiple areas simultaneously.
One of the advantages EBM has over LPBF is its ability to handle conductive materials and reflective metals, such as copper. Another feature of EBM is its ability to nest or stack separate parts within the build chamber, as they don’t necessarily have to be attached to the build plate, significantly increasing volume output.
Compared to lasers, electron beams usually produce thicker layers and rougher surface features. Due to the high temperature in the build chamber, EBM printed parts may not require post-print heat treatment to eliminate stress.
Material jetting is a 3D printing process where tiny droplets of material are deposited and then solidified or cured on a build plate. This process uses a photosensitive polymer or wax droplets that solidify when exposed to light, constructing objects one layer at a time.
The nature of the material jetting process allows for different materials to be printed on the same object. An application of this technology is the manufacturing of parts with varying colors and textures.
The Material Jetting (M-Jet) of polymers is a 3D printing process where a layer of photosensitive resin is selectively deposited onto a build plate and cured using ultraviolet (UV) light.
After a layer is deposited and cured, the build platform lowers by a layer thickness, and the process is repeated to build a 3D object. M-Jet combines the high precision of resin 3D printing with the speed of filament 3D printing (FDM) to create parts and prototypes with realistic colors and textures.
All material jetting 3D printing technologies are not entirely identical. Differences exist between printer manufacturers and proprietary materials. M-Jet machines deposit build materials in a line-by-line manner from multiple rows of print heads.
This method allows the printer to manufacture multiple objects on one line without impacting build speed. As long as the model is correctly arranged on the build platform, and space within each build line is optimized, M-Jet can produce parts faster than many other types of resin 3D printers.
Objects manufactured with M-Jet require support, which is simultaneously printed with dissolvable material during the build process and removed in the post-processing stage. M-Jet is one of the few 3D printing technologies that offer objects made from multi-material printing and full color.
Material jetting machines are not available in hobbyist versions; these machines are more suitable for professionals in car manufacturing, industrial design firms, art studios, hospitals, and all types of product manufacturers who want to create accurate prototypes to test concepts and bring products to the market faster.
Unlike vats polymerization technology, M-Jet does not require post-curing as UV light in the printer fully cures each layer.
Aerosol Jetting
Optomec developed the Aerosol Jet, a unique technology primarily used for 3D printing electronic products. Resistors, capacitors, antennas, sensors, and Thin-Film Transistors are all printed using Aerosol Jet technology. It can be roughly compared to spray painting, but it differs from industrial coating processes in that it can be used to print complete 3D objects.
Electronic ink is placed into an atomizer, which produces droplets with diameters between 1 and 5 microns. The aerosol mist is then delivered to the deposition head, focused by a sheath gas, creating a high-speed particle spray.
As the entire process uses energy, this technology is sometimes also called Directed Energy Deposition, but since the material in this case is in droplet form, we include it in material jetting.
Freeform Plastic Molding
German company Arburg created a technology called freeform plastic molding (APF), a combination of extrusion and material jetting technologies. It uses commercially available plastic granules, which are melted in the injection molding process and moved to the discharge unit.
Fast opening and closing motion of the high-frequency nozzle, producing up to 200 plastic droplets per second with diameters between 0.2 and 0.4 mm. The droplets bond with the solidifying material during cooling. Post-processing is generally not required. If support material was used, it must be removed.
NanoParticle Jetting (NPJ) is one of the few proprietary technologies that are difficult to classify, developed by a company named XJet. It uses a printhead array with thousands of inkjet nozzles that can simultaneously jet millions of ultra-fine material droplets onto a super-thin layer of the build tray, while also jetting support material.
Metal or ceramic particles are suspended in the liquid. The process happens at high temperatures, where the liquid evaporates when jetted, leaving mostly metal or ceramic material. The resulting 3D parts only have a small amount of binding agent left, which is removed in the sintering post-processing.
Binder jetting is a 3D printing process that selectively bonds a layer of powder in specific areas using a liquid adhesive. This type of technology combines the characteristics of powder bed fusion and material jetting.
Similar to PBF, binder jetting uses powdered material (metals, plastics, ceramics, wood, sugar, etc.) and like material jetting, liquid adhesive polymer is deposited from an inkjet. The process of binder jetting remains the same whether it’s metal, plastic, sand or other powdered materials.
Firstly, a coating blade smears a thin layer of powder on the build platform. Then, a print head equipped with an inkjet nozzle passes over the bed, selectively depositing droplets of adhesive to bond the powder particles together. Once a layer is complete, the build platform moves downward and the blade re-coats the surface. This process is repeated until the entire piece is finished.
The uniqueness of binder jetting lies in the absence of heat during the printing process. The adhesive acts as a glue holding the polymer powder together. After printing, the part is encased in unused powder, which is typically left to solidify. The piece is then removed from the powder bin, excess powder is collected and can be reused.
From here, post-processing is required depending on the material, except for sand, which can usually be used directly from the printer as a core or mold. When the powder is metal or ceramic, heat-involved post-processing melts away the adhesive, leaving only the metal. Plastic part post-processing usually includes coating to improve surface smoothness. Polishing, painting, and sanding can also be done on polymer binder jetting parts.
Binder jetting is fast and has a high production rate, hence, compared to other AM methods, it can produce a large number of parts more cost-effectively. Metal binder jetting is applicable for a variety of metals and is popular in final use consumer goods, tools, and batch spare parts.
However, the material selection for polymer binder jetting is limited and the structural performance of the parts produced is lower. Its value lies in its ability to create full-color prototypes and models.
Binder Jetting can also be used to manufacture solid metal objects with complex geometrical shapes, far beyond the capability of traditional manufacturing technologies. Metal binder jetting is a very attractive technology for mass-producing metal parts and achieving light-weighting.
As binder jetting can print parts with complex pattern infills rather than solids, the resulting parts are significantly lighter but retain their strength. The porosity characteristics of binder jetting can also be used to create lighter end parts for medical applications, such as implants.
In general, the material performance of metal binder jetted parts is comparable to metal parts produced with metal injection molding, one of the most widely used manufacturing methods in mass production of metal parts. Additionally, binder jetted parts exhibit higher surface smoothness, especially in internal channels.
Metal binder jetted parts need secondary processing after printing to achieve good mechanical properties. Fresh out of the printer, the parts essentially consist of metal particles held together by a polymer binder.
These so-called “green parts” are too fragile to be used as-is. After the printed parts are removed from the metal powder bed (a process called depowdering), they undergo heat treatment (a process called sintering) in a furnace.
Both the printing parameters and sintering parameters are adjusted for the specific geometry, material, and required density of the part. Sometimes bronze or other metals are used to infiltrate the voids in binder jetted parts, thereby achieving zero porosity.
Plastic binder jetting is a process very similar to metal binder jetting as it also uses powder and liquid binder, but the applications are quite different. Once printing is complete, plastic parts are removed from their powder bed and cleaned, usually ready for use without further processing, but these parts lack the strength and durability found in other 3D printing processes.
Plastic binder jetted parts can be infused with another material to increase their strength. Binder jetting with polymers is favored for its ability to produce multicolored parts for medical modeling and product prototyping.
Sand adhesive jetting differs from plastic adhesive jetting in terms of the printing machine and process used, hence the separation. One of the most common uses of adhesive jetting technology is the production of large sand casting molds, models, and cores. The low cost and speed of this process make it an excellent solution for foundries, as it is challenging to produce intricate pattern designs within hours using traditional technologies.
The future of industrial development is constantly making high demands on contractors and suppliers. Sand 3D printing is just beginning to tap into its potential. After printing, the operator needs to remove the cores and molds from the build area and clean them to remove any loose sand. The molds can usually be prepared for casting immediately. After casting, the mold is broken open, and the final metal part is removed.
Another unique and brand-specific 3D printing process that doesn’t easily fit into any existing category, and is not actually adhesive jetting, is HP’s Multi Jet Fusion. MJF is a polymer 3D printing technology that uses powdered material, liquid fusion material, and a detailing agent.
It is not considered adhesive jetting because heat is added in this process, producing parts with greater strength and durability, and the liquid is not entirely an adhesive. The name of this process comes from the multiple inkjet heads used in the printing process.
During the Multi Jet Fusion printing process, the printer lays a layer of material powder, usually nylon, on the print bed. After this, the inkjet heads pass over the powder and deposit the fusion agent and detailing agent on it. An infrared heating device then moves over the print. Wherever the fusion agent is added, the underlying layers melt together, while areas with the detailing agent remain powdery.
The powdery parts fall off, producing the desired geometric shape. This also eliminates the need for modeling support, as the lower layers support the layers printed on top of them. To complete the printing process, the entire powder bed and the printed parts within it are moved to a separate processing station, where most of the loose, unmelted powder is vacuumed away for reuse.
Multi Jet Fusion is a versatile technology that has been applied in various industries, including automotive, healthcare, and consumer goods.
Directed Energy Deposition (DED) is a 3D printing process where metal material is supplied and melted simultaneously with a powerful energy supply. It’s one of the broadest categories of 3D printing, encompassing many subcategories, depending on the form of material (wire or powder) and type of energy (laser, electron beam, arc, supersonic, thermal, etc.). Essentially, it has many similarities with welding.
This technology is used for layer-by-layer printing, usually followed by CNC machining to achieve tighter tolerances. The combination of DED and CNC is very common, with a subtype of 3D printing called Hybrid 3D Printing, which includes DED and CNC units in the same machine.
This technology is considered a faster, cheaper alternative to small batch metal casting and forging, and a key repair for applications in the offshore oil and gas industry, as well as aerospace, power generation, and utility sectors.
Laser Directed Energy Deposition (L-DED), also known as Laser Metal Deposition (LMD) or Laser Engineered Net Shaping (LENS), uses metal powder or wire delivered through one or more nozzles and melted onto a build platform or metal part by a powerful laser. As the nozzle and laser move, or the part moves on a multi-axis turntable, the object is built up layer by layer.
The build speed is faster than powder bed fusion, but results in reduced surface quality and significantly reduced accuracy, typically requiring substantial post-processing. Laser DED printers usually have a sealed chamber filled with argon gas to prevent oxidation. When handling less reactive metals, they can operate using only local argon or nitrogen gas.
Common metals used in this process include stainless steel, titanium, and nickel alloys. This printing method is typically used to repair high-end aerospace and automotive parts, such as jet engine blades, but is also used to produce entire parts.
Electron Beam DED, also known as Wire Electron Beam Energy Deposition, is a 3D printing process very similar to Laser DED. It is conducted in a vacuum chamber and can produce very clean, high-quality metals. As a metal wire passes through one or more nozzles, it is melted by an electron beam.
The layers are built individually, with the electron beam forming a small melt pool into which the wire is fed by a wire feeder. When handling high-performance and active metals (such as copper, titanium, cobalt, and nickel alloys), the electron beam is chosen for DED.
DED machines are effectively unlimited in terms of print size. For example, 3D printer manufacturer Sciaky has an EB DED machine capable of producing parts nearly 6 meters long at a rate of 3 to 9 kilograms of material per hour.
Electron Beam DED is touted as one of the fastest methods for manufacturing metal parts, although it is not the most precise, making it an ideal machining technology for building large structures (such as airframes) or replacement parts (such as turbine blades).
Wire Directed Energy Deposition, also known as Wire Arc Additive Manufacturing (WAAM), is a form of 3D printing that uses energy in the form of plasma or an electric arc to melt metal in wire form, which is then deposited layer by layer onto a surface, such as a multi-axis turntable, by a robotic arm to form a shape.
This method is chosen over similar technologies using lasers or electron beams because it does not require a sealed chamber and can use metals identical to those used in traditional welding (sometimes even the exact same material).
Electric Direct Energy Deposition is considered the most cost-effective choice in DED technology as it can use existing arc welding robots and power sources, making the barrier to entry relatively low.
Unlike welding, however, this technique uses complex software to control a variety of variables during the process, including heat management and tool paths of the robotic arm. This technique does not have support structures to be removed, and finished parts are commonly CNC machined when necessary to achieve strict tolerances or surface polishing.
Cold Spray Coating is a DED 3D printing technology that uses supersonic spraying of metal powder to bond them together without melting, virtually eliminating thermal cracking or stress.
Since the early 2000s, it has been used as a coating process, but recently several companies have begun using cold spray coating for additive manufacturing because it can print at speeds 50 to 100 times faster than typical metal 3D processes and does not require inert gas or a vacuum chamber.
Like all DED processes, Cold Spray Coating does not produce prints with good surface quality or detail, but parts can be used directly off the print bed.
Melt Directed Energy Deposition is a 3D printing process that uses heat to melt metal (usually aluminum), which is then deposited layer by layer onto a build plate to form a 3D object. The difference between this technology and metal extrusion 3D printing is that extrusion uses metal feedstock with a small amount of polymer inside, allowing the metal to be extruded.
The polymer is then removed in a heat treatment stage, whereas Melt DED uses pure metal. Melt or liquid DED can also be likened to material jetting, but instead of a series of nozzles depositing droplets, liquid metal typically flows out of a nozzle.
Variations of this technology are being developed, and Melt Metal 3D printers are rare. The advantage of using heat to melt and then deposit metal is that it uses less energy than other DED processes and can potentially use recycled metal as feedstock, rather than metal wire or highly processed metal powder.
Sheet Lamination is technically a form of 3D printing, but it significantly differs from the aforementioned techniques. Its function is to stack and laminate very thin material sheets together to produce 3D objects or stacks, which are then cut mechanically or by laser to form the final shape.
The material layers can be fused together using various methods, including heat and sound, depending on the material, with materials ranging from paper and polymers to metals. When parts are laminated and then laser cut or machined into the desired shape, more waste is generated than with other 3D printing technologies.
Manufacturers use thin sheet lamination to produce non-functional prototypes at a relatively high speed and cost-effectively, suitable for battery technology and the production of composite materials, as the materials used can be interchanged during the printing process.
Lamination is a form of 3D printing technology where material sheets are stacked together and glued, and then the layered object is cut into the correct shape using a knife (or laser or CNC router). This technology is less common today as the cost of other 3D printing technologies has decreased, and their speed and ease of use have significantly increased.
Viscous Lithography Manufacturing (VLM): VLM is BCN3D’s patented 3D printing process that laminates thin layers of high-viscosity photosensitive resin onto a transparent transfer film. The mechanical system allows resin to be laminated from both sides of the film, enabling the combination of different resins to obtain multi-material parts and easily removable support structures. This technology has not been commercialized yet, but it could be considered a type of laminated 3D printing technology.
Composite-Based Additive Manufacturing (CBAM): Start-up Impossible Objects has patented this technology, which fuses carbon, glass, or Kevlar pads with thermoplastic plastic to manufacture parts.
Selective Lamination Composite Material Manufacturing (SLCOM): EnvisionTEC, now known as ETEC and owned by Desktop Metal, developed this technology in 2016, which uses thermoplastic plastic as the base material and woven fiber composite material.
Note: There are many types of 3D printing technologies; the above are the seven most common types of additive manufacturing technologies in 3D printing and do not cover all 3D printing technologies on the market.