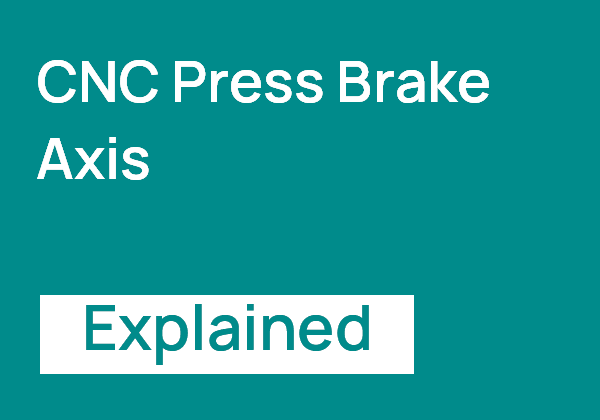
Ever wondered how sheet metal is expertly bent into intricate shapes? This article explores six types of press brake bending processes—folding, wiping, air bending, bottoming, coining, and three-point bending. You’ll learn the unique benefits and limitations of each method, and how they contribute to efficient and precise metal fabrication. From simple bends to complex profiles, these techniques are essential for producing high-quality parts in manufacturing. Dive in to understand how these bending processes can enhance your projects.
Press brakes are versatile machines capable of producing high-quality parts, but challenges remain in achieving optimal results. This discussion will explore various bending types and the key factors influencing the press brake process.
A reliable and reproducible press brake operation requires a synergistic combination of the machine itself and its tooling components.
The typical press brake configuration consists of two robust C-frames forming the machine’s sides, connected by a substantial table at the bottom and a movable upper beam at the top. Alternatively, an inverted configuration is also possible.
The bottom die rests on the table, while the top punch attaches to the upper beam. In hydraulic press brakes, which dominate current production, the upper beam is actuated by two synchronized hydraulic cylinders mounted on the C-frames.
Press brake capabilities are defined by several critical parameters, including:
The upper beam typically operates at speeds ranging from 1 to 15 mm/sec, depending on the application requirements.
Modern press brakes increasingly incorporate multi-axis computer-controlled backgauges and advanced sensor systems for real-time process optimization. These sensors, both mechanical and optical, measure the bending angle during the forming cycle and transmit data to the machine controls. This feedback loop allows for dynamic adjustments of process parameters, ensuring consistent part quality.
The press brake bending process is a complex interplay of several factors:
1. Top tool (punch) geometry:
2. Bottom tool (die) geometry:
3. Machine parameters:
During the folding process, the longest leg of the sheet metal workpiece is securely clamped between two precision-aligned clamping beams. The bend beam then ascends or descends, folding the extending portion of the sheet around a carefully selected bend profile, as illustrated in Figure 1.
State-of-the-art bending machines feature versatile bend beams capable of executing both upward and downward forming operations. This bi-directional capability offers a significant advantage when fabricating complex components that require a combination of positive and negative bend angles, enhancing production flexibility and reducing setup times.
The final bend angle is determined by the interplay of several critical factors: the folding angle of the bending beam, the specific geometry of the tooling (including the bend profile radius and die opening), and the inherent material properties of the sheet metal (such as yield strength, elastic modulus, and strain hardening characteristics).
Folding as a bending technique offers notable advantages in handling large sheet metal panels with relative ease, making it particularly amenable to automation in high-volume production environments. The process minimizes direct contact between tooling and the sheet surface, significantly reducing the risk of marring or scratching, which is crucial for maintaining surface quality in visible or aesthetically important components.
However, a key consideration in folding operations is the spatial requirement and cycle time associated with the bend beam’s movement. The need for sufficient clearance to accommodate the beam’s travel path can impact machine footprint and may limit throughput in certain applications. Engineers must carefully balance these factors against the process’s benefits when designing production workflows.
In the wiping process, the sheet metal is securely clamped between the upper and lower clamping beams of the press brake. The wiping die, typically attached to the machine’s ram, then descends to bend the protruding portion of the sheet around the lower die’s radius, as illustrated in Figure 2. This action creates a controlled, sweeping motion that forms the desired bend.
Compared to air bending or bottoming, wiping is a more rapid technique for creating bends, offering increased productivity for high-volume production. However, this speed comes with an elevated risk of surface marring or damage to the workpiece. As the wiping die slides over the sheet’s surface during the bending action, it can potentially cause scratches or other imperfections, particularly when forming sharp angles or working with materials that have sensitive finishes.
Wiping is predominantly employed in the fabrication of panel-type products featuring small profiled edges, such as electrical enclosures, HVAC ductwork, and architectural panels. The technique is especially effective for creating narrow flanges and hem bends. With specialized tooling, wiping operations can be efficiently performed on standard press brakes, making it a versatile option for many sheet metal fabricators.
To mitigate the risk of surface damage during wiping, several strategies can be employed:
These measures help maintain product quality while leveraging the speed advantages of the wiping process.
When it comes to sheet metal bending, there are four primary variations: air bending, bottoming, coining, and three-point bending. Each method offers distinct advantages and is suited for specific applications based on material properties, required accuracy, and production volume.
The fundamental characteristic of bending is that the sheet metal is pressed by a top tool (punch) into the opening of the bottom tool (die), as illustrated in Figure 3. This process induces plastic deformation in the material, creating a permanent bend along a predetermined line.
As a result of the bending process, sheet metal on each side of the bend experiences elastic spring-back and is lifted, which can cause issues such as sagging and folding, particularly with large or thin sheets. These challenges are more pronounced when dealing with high-strength materials or complex geometries.
To address these issues, alternative forming techniques such as folding or wiping are often preferred for larger components. Additionally, sheet follow supports or back gauges can be integrated with the press brake to mitigate lifting and improve accuracy. These supporting tools ensure consistent bend angles and reduce the risk of material distortion.
When bending involves both positive and negative angles in the same component, folding machines offer greater flexibility than traditional press brake techniques. Folding allows for more complex bend sequences and can handle larger sheet sizes without compromising precision.
One of the significant advantages of using modern CNC press brakes is the increased speed, flexibility, and repeatability they offer. These machines can quickly switch between different tooling setups, accommodate various bend angles, and execute complex bending sequences with minimal operator intervention. This versatility makes press brakes ideal for both small batch production and high-volume manufacturing scenarios.
Air bending, also known as partial bending, is a versatile and widely adopted sheet metal forming technique that has gained prominence due to significant advancements in press brake technology. This method offers superior control over springback, making it the preferred choice for many fabricators.
In air bending, the sheet metal is pressed into a V-shaped die by a punch, creating a bend without full contact between the workpiece and the tooling. The process derives its name from the air gap maintained between the sheet and the die during forming. Contact occurs at only three points: two along the die shoulders and one at the punch tip, hence the term “three-point bending.”
The bending process is achieved by lowering the punch to a predetermined depth within the V-die opening, without bottoming out. This partial contact allows for greater flexibility in producing various bend angles and profiles using a single set of tooling. The bend angle is primarily controlled by the punch stroke depth, rather than the tooling geometry.
One of the key advantages of air bending is its adaptability. A single tool set can accommodate multiple material thicknesses and types, as well as a range of bend angles. This versatility significantly reduces tool change-over times, enhancing overall productivity. Additionally, air bending requires less bending force compared to other methods, allowing for the use of smaller, more compact tools and providing greater design flexibility.
The V-die opening width is a critical parameter in air bending, typically expressed as a multiple of the sheet thickness (S). For thin sheets up to 3 mm, a width of 6S is common, while thicker sheets over 10 mm may require up to 12S. A general rule of thumb is V = 8S, though this can vary based on specific application requirements.
Despite its advantages, air bending has some limitations. The process is generally less precise than methods involving full sheet-to-tool contact throughout the bend. Accuracy is highly dependent on the consistency of material properties, sheet thickness, and tooling condition. Variations in these factors can lead to deviations in the final bend angle due to springback effects.
Typical angle accuracy for air bending is approximately ±0.5 degrees. The bend radius is not directly determined by the tool shape but is influenced by material elasticity, usually falling between 1S and 2S. To mitigate quality issues arising from material and tooling variations, fabricators often employ advanced technologies such as real-time angle measurement systems, adaptive crowning systems, and wear-resistant tooling.
The reduced tonnage requirements and high flexibility of air bending have made it increasingly popular among modern fabricators. However, to fully leverage its benefits, careful consideration must be given to material properties, tooling design, and process control. By implementing appropriate compensatory measures and leveraging the latest press brake technologies, manufacturers can achieve high-quality, consistent results with air bending across a wide range of applications.
Advantages:
Disadvantages:
Bottoming is an advanced variation of air bending that involves pressing a sheet against the slopes of the V-opening in the bottom tool (Fig. 5), while trapping air between the sheet and the V-opening’s bottom. This process offers superior precision and consistency compared to air bending, making it ideal for creating precise profiles.
In bottoming, the punch descends to the die vee’s bottom, pressing the sheet metal firmly against the die sides. This concentrated pressure in the bend area yields a more accurate internal radius and increased material yielding, resulting in reduced springback.
The selection of tooling is critical in bottoming. Operators must carefully choose the optimal angles for both punch and die, considering the expected springback to achieve the desired profile angle. For optimal results, the punch and die angles must match precisely.
Unlike air bending, bottoming offers less flexibility as the punch radius and V-opening angle are directly linked. This necessitates separate tool sets for each bend angle, sheet thickness, and often for different materials due to variations in springback and required tool compensation.
The ideal V-opening width (U-shaped openings are not suitable) for bottoming follows a general guideline:
Minimum acceptable bending radii for sheet steel typically range from 0.8S to 2S, depending on material quality. Softer materials like copper alloys can achieve much smaller radii, with a lower limit of 0.25S possible under optimal conditions.
Force requirements for bottoming vary:
The resulting bend angle is predominantly determined by the tooling, with only springback requiring correction. Notably, bottoming generally results in less springback compared to air bending. Theoretically, bottoming can achieve angle accuracies as precise as ±0.25 degrees.
However, it’s important to note that recent advancements in press brake control and adjustment capabilities, even in more affordable machines, have led to air bending becoming increasingly preferred over bottoming in many applications. This shift is due to air bending’s improved precision and greater flexibility, which can now often match or exceed the benefits traditionally associated with bottoming.
Advantages:
Disadvantages:
Coining, derived from the process of minting metal coins, is a precision bending technique that achieves highly accurate and consistent results in sheet metal forming. This method is characterized by its ability to produce identical parts with minimal springback, making it ideal for applications requiring extreme precision.
In coining, the punch and die angles are identical to the desired bend angle, eliminating the need for springback compensation. The process involves applying substantial force—typically 4 to 5 times that of air bending—to permanently deform the material throughout its entire cross-section. This high-pressure forming technique can require up to 25 to 30 times more tonnage than air bending in some cases, necessitating robust press brakes and tooling.
The die opening in coining is notably narrower than in air bending or bottoming, ideally measuring about five times the sheet metal thickness (5T). This reduced width prevents excessive material flow and maintains tight control over the internal radius of the bend. The punch tip penetrates the material significantly, creating a precise, permanent deformation that virtually eliminates springback.
Key characteristics of coining include:
While coining offers unparalleled precision, its high force requirements and potential for tool wear make it more expensive than air bending or bottoming. Consequently, it is typically reserved for thin sheets and applications where extreme accuracy is paramount.
The coining process is illustrated in Figure 6, showing the punch fully engaged with the material, forcing it to conform precisely to the die opening’s bottom profile.
Advantages:
Disadvantages:
Three-point bending is an advanced bending technique that has gained traction in precision metalworking, often considered a sophisticated evolution of air bending.
This method employs a specialized die system where the bottom tool’s height is precisely controlled via a servo motor, offering micron-level adjustability (typically ±0.01 mm). The sheet metal is formed over the die’s bend radii until it contacts the adjustable bottom, with the bend angle inversely proportional to the depth of the die bottom.
To ensure exceptional accuracy, the process incorporates a hydraulic cushion between the ram and the upper tool. This dynamic compensation system adjusts for variations in sheet thickness, allowing for real-time corrections during the bending operation. Consequently, three-point bending can achieve bend angles with a precision of less than 0.25 degrees, surpassing the capabilities of conventional bending methods.
The key advantages of three-point bending include:
However, the adoption of this technique faces certain challenges:
Given these factors, three-point bending is currently most prevalent in high-value, precision-driven sectors such as aerospace, medical device manufacturing, and advanced electronics. In these niche markets, the enhanced accuracy and repeatability justify the additional costs, particularly for complex parts with stringent geometric requirements.
As the technology matures and becomes more accessible, it’s anticipated that three-point bending will find broader applications in metal fabrication, especially as industries increasingly demand higher precision and flexibility in sheet metal forming processes.