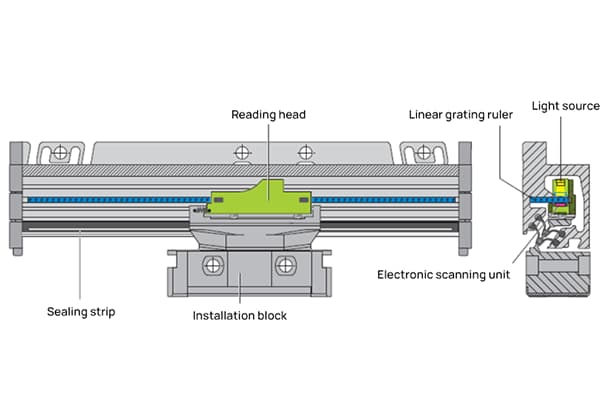
Have you ever marveled at the versatility of springs in mechanical engineering? From automotive suspensions to precision instruments, these unsung heroes play a crucial role. In this blog post, we’ll delve into the fascinating world of springs, exploring their types, applications, and design principles. Join us as we uncover the secrets behind these essential components that keep our machines and devices running smoothly.
Cylindrical helical compression springs with circular cross-sections are characterized by their linear force-displacement relationship, consistent stiffness throughout their range of motion, and simple yet effective design. These attributes, combined with ease of manufacture and cost-effectiveness, contribute to their widespread adoption across various industries.
These springs excel in applications requiring:
Under equivalent space constraints, a rectangular section cylindrical helical compression spring demonstrates superior mechanical properties compared to its circular section counterpart. This design exhibits significantly higher stiffness and enhanced energy absorption capacity, making it particularly advantageous in applications where space is limited but performance demands are high.
The rectangular cross-section offers several key benefits:
Cylindrical helical compression springs with flat wire sections offer significant advantages over their circular-section counterparts, particularly in terms of energy storage capacity, compression height, and overall compression capability. The flat wire profile allows for a more efficient use of material and space, resulting in springs that can store more potential energy within a given volume.
These springs exhibit a lower solid height (fully compressed height) due to the reduced gap between coils when compressed, enabling a greater range of motion within confined spaces. Additionally, the flat wire geometry provides increased surface area contact between coils, enhancing stability and load distribution during compression.
The superior performance characteristics of flat section springs make them ideal for applications with stringent space constraints and high energy requirements. They are widely employed in automotive engineering, finding extensive use in:
As the applied load increases to a critical threshold, the spring’s behavior undergoes a distinctive transformation. The coils with smaller pitch progressively compress and come into contact, a phenomenon known as coil binding. This gradual engagement of coils results in a non-linear increase in spring stiffness, causing the force-deflection characteristic to transition from an initial linear response to a progressive, incremental curve.
The variable stiffness characteristic inherently alters the spring’s natural frequency as a function of load, creating a dynamic system with adaptive resonant properties. This feature enables the spring to effectively attenuate or mitigate resonance across a broader spectrum of operating frequencies, particularly beneficial in high-speed applications subject to rapidly fluctuating loads.
The unique load-dependent behavior of unequal pitch springs makes them especially valuable in precision mechanisms, automotive suspensions, and industrial machinery where controlled energy absorption and vibration isolation are crucial. By tailoring the pitch variation along the spring’s length, engineers can fine-tune the force-deflection profile to meet specific performance requirements, optimizing the spring’s response to complex loading scenarios.
The multi-strand cylindrical helical compression spring utilizes a composite material consisting of steel wire rope intricately twisted with thin steel wire filaments. This unique construction combines the strength of wire rope with the flexibility of individual wire strands.
In its unloaded state, the contact between the wires within the rope structure remains relatively loose, allowing for initial compliance. However, as the external load increases to a critical threshold, a significant transformation occurs. The individual wire strands begin to tighten and compress against each other, resulting in a marked increase in spring rigidity. This phenomenon manifests as a distinct inflection point in the force-displacement characteristic curve of the multi-strand helical spring, differentiating it from conventional single-wire springs.
The multi-strand design offers several advantages over traditional cylindrical helical springs fabricated from equivalent cross-sectional materials:
The performance and characteristics of cylindroid helical-coil extension springs closely parallel those of cylindrical helical compression springs with circular cross-sections. However, they are specifically designed to operate under tensile loads rather than compressive forces.
These springs are primarily utilized in applications that require controlled tensile loading and energy storage. Common use cases include:
The unique geometry of cylindroid helical-coil extension springs, featuring a gradually increasing coil diameter along the spring axis, provides several advantages:
A cylindrical helical torsion spring is an essential mechanical component engineered to resist rotational forces and store torsional energy. Its helical coil structure, typically wound from high-strength materials like spring steel or nickel alloys, enables it to efficiently convert applied torque into potential energy. This spring type exhibits a linear force-deflection characteristic, making it predictable and reliable in various applications.
Commonly utilized in diverse industrial and consumer products, cylindrical helical torsion springs serve multiple functions:
The conical coil spring exhibits a unique functionality akin to that of an unequal pitch coil spring, but with enhanced performance characteristics. As the applied load increases, the spring undergoes a progressive tightening process, initiating from the larger diameter coils and advancing towards the smaller diameter coils. This gradual engagement continues until the coils make contact, resulting in a variable spring rate.
The force-deflection characteristic of a conical coil spring is distinctly non-linear, with stiffness increasing progressively as the load increases. This variable stiffness profile leads to a dynamic natural vibration frequency, which is particularly advantageous for mitigating or eliminating resonance effects. Compared to conventional equal pitch compression springs, conical springs demonstrate superior anti-resonance capabilities across a broader frequency spectrum.
The tapered geometry of conical springs offers several key benefits:
These attributes make conical coil springs particularly well-suited for applications requiring heavy load support and vibration attenuation. Common industrial applications include:
Volute coil springs, characterized by their conical shape and tightly wound coils, offer superior energy absorption capacity in a compact form factor compared to conventional helical springs. This unique design allows for greater deflection and load-bearing capabilities within a limited space. Moreover, the inter-plate friction inherent in their structure provides significant damping effects, effectively attenuating vibrations across a wide frequency spectrum.
These springs find extensive application in industrial piping systems, particularly where thermal expansion compensation and vibration isolation are critical. Their implementation is especially prevalent in high-temperature and high-pressure environments, such as steam and water distribution networks in thermal power plants. The springs’ ability to accommodate axial, lateral, and angular movements makes them invaluable in maintaining system integrity and prolonging equipment lifespan.
Despite their advantages, volute coil springs present certain manufacturing and maintenance challenges. The narrow inter-plate gaps inherent to their design complicate heat treatment processes, making uniform quenching difficult to achieve. This can lead to inconsistencies in material properties and performance. Additionally, the tightly wound structure precludes conventional surface treatment methods like shot blasting, potentially limiting corrosion resistance and fatigue life. Furthermore, the complex geometry of volute springs demands high-precision manufacturing techniques, which can be challenging to maintain consistently, potentially affecting the spring’s load-deflection characteristics and overall reliability.
To mitigate these limitations, advanced manufacturing technologies such as computer-controlled coiling machines and specialized heat treatment processes are being developed. These innovations aim to enhance production accuracy, improve material uniformity, and explore alternative surface treatment methods suitable for the unique geometry of volute coil springs.
The design principle of torsion bar springs is conceptually straightforward, but their production demands exacting material specifications and manufacturing precision. These springs operate on the principle of torsional elasticity, where a metal bar resists twisting forces and returns to its original position when the load is removed.
Torsion bar springs find widespread application in automotive suspension systems, particularly in light vehicles and some heavy-duty trucks. They offer a compact design that saves space and provides a progressive spring rate, enhancing ride comfort and handling. In the automotive sector, they are often made from high-strength alloy steels, such as SAE 5160 or 9260, heat-treated to achieve optimal mechanical properties.
Beyond automotive use, torsion bars serve as auxiliary springs in various mechanical systems. In internal combustion engines, they are employed in valve assemblies to ensure precise valve timing and sealing. Air spring systems in industrial and transportation applications may incorporate torsion bars to provide additional stability and load distribution. Pressurizers in hydraulic and pneumatic systems also benefit from torsion bar springs, where they help maintain consistent pressure levels.
The manufacturing process for torsion bars typically involves precision forging or machining, followed by carefully controlled heat treatment to achieve the required strength and elasticity. Surface treatments like shot peening or nitriding are often applied to enhance fatigue resistance and durability. Quality control measures, including non-destructive testing and rigorous dimensional checks, are crucial to ensure consistent performance and longevity in these critical components.
Belleville springs, also known as coned-disc springs or belleville washers, exhibit exceptional load-bearing capacity, cushioning properties, and shock absorption characteristics. These conical spring washers are designed to support high loads in a compact axial space, making them ideal for applications with limited installation height.
By utilizing different combinations and stacking arrangements of Belleville springs, engineers can achieve a wide range of load-deflection characteristics. These configurations include:
The versatility of Belleville springs allows for their implementation in numerous industrial applications, such as:
Ring springs, also known as friction springs or annular springs, are sophisticated energy absorption devices widely employed in heavy-duty industrial and military applications. These springs excel in scenarios demanding high energy dissipation within compact spaces, offering superior performance compared to conventional helical springs. Their unique design consists of a series of inner and outer rings with tapered mating surfaces, allowing for controlled friction and energy absorption during compression and expansion cycles.
Key applications of ring springs include:
The plane volute spring, also known as a hairspring, is a precision-engineered component consisting of a flat, tightly coiled metal strip. This versatile spring serves dual functions in various mechanical systems:
The primary function of the plane volute spring is energy storage and controlled release. Its unique coiled design allows for efficient energy accumulation and gradual dissipation, making it ideal for timekeeping mechanisms.
Key advantages of plane volute springs in clockwork systems include:
Applications of plane volute springs extend across various industries and products:
Leaf springs are versatile elastic components consisting of one or more layers of metal sheets, typically with a rectangular cross-section. While traditionally associated with vehicle suspension systems, they find diverse applications in various industrial and mechanical contexts, particularly where controlled flexibility and load-bearing capacity are required.
These springs excel in scenarios demanding moderate load capacity and predictable deformation characteristics. Their unique design allows for a wide range of applications, including:
The steel plate spring, also known as a leaf spring, comprises multiple layers of high-strength spring steel plates, typically varying in length and thickness.
This robust component is widely employed in the suspension systems of various vehicles, including automobiles, heavy-duty trucks, tractors, and railway cars. Its primary function is to absorb and distribute shock loads, provide cushioning, and minimize vibrations, thereby enhancing ride comfort and vehicle stability.
Beyond its traditional automotive applications, the steel plate spring finds extensive use as a vibration dampening device in numerous mechanical products and industrial equipment. Its high rigidity, coupled with excellent fatigue resistance and load-bearing capacity, makes it an ideal choice for applications requiring both strength and flexibility. Examples include heavy machinery, agricultural equipment, and certain types of industrial presses.
Rubber springs, characterized by their low elastic modulus, exhibit exceptional elastic deformation capabilities, enabling the achievement of desired nonlinear characteristics with remarkable ease. This unique property allows for a wide range of design flexibility, as rubber springs can be manufactured in virtually any shape and with customizable stiffness to meet specific load requirements in multiple directions.
One of the key advantages of rubber springs is their ability to simultaneously bear multi-axial loads, including compression, tension, shear, and torsion. This multi-functional capacity significantly simplifies overall system designs, reducing the need for complex assemblies and potentially lowering component count and weight.
The versatility of rubber springs extends to their material composition, with various elastomer compounds available to suit different environmental conditions and performance requirements. For instance, natural rubber offers excellent elasticity and fatigue resistance, while synthetic rubbers like EPDM provide enhanced weather and chemical resistance.
Recent advancements in rubber compound technology and manufacturing processes have further expanded the application scope of rubber springs. Improved durability, temperature resistance, and load-bearing capacity have led to their increasing adoption in diverse industries, including automotive suspension systems, vibration isolation in industrial machinery, and seismic protection for buildings.
The rapid growth in the use of rubber springs in mechanical equipment can be attributed to several factors:
The rubber-metal spiral composite spring exhibits a progressive characteristic curve, demonstrating increasing stiffness under load. This non-linear behavior provides adaptive performance across various loading conditions.
This innovative composite design combines the best properties of both rubber and metal springs. Compared to a conventional rubber spring, it offers significantly enhanced rigidity and load-bearing capacity. In contrast to a traditional metal spring, it provides superior damping characteristics and energy absorption.
Key advantages of the rubber-metal spiral composite spring include:
An air spring is an advanced pneumatic suspension component that harnesses the compressibility of gas, typically air or nitrogen, to provide a highly responsive and adjustable elastic effect. This non-metallic spring technology offers several advantages over traditional metal springs in vehicle suspension systems, significantly enhancing dynamic performance, ride comfort, and load-carrying capacity.
Key features and benefits of air springs include:
Air spring technology has found widespread adoption in various transportation applications, including:
It is utilized to assess non-linear quantities related to pressure, including the flow of liquids or gases in pipelines and the speed and altitude of aircraft.
It is utilized as the sensitive component in the instrument, serving to isolate two distinct substances. For instance, it functions as a flexible sealing device that deforms in response to changes in pressure or vacuum.
Two identical diaphragms are connected into a box shape along the periphery.
The pressure spring tube, also known as a Bourdon tube, is a critical sensing element in numerous pressure measurement instruments. When subjected to fluid pressure, the sealed end of this curved, elliptical cross-section tube undergoes elastic deformation, resulting in a measurable displacement. This displacement is precisely transmitted to a pointer or digital display through a finely-tuned mechanical linkage or electronic transducer.
The principle of operation relies on the tube’s geometry: as internal pressure increases, the tube tends to straighten, with the magnitude of movement directly proportional to the applied pressure. This simple yet effective mechanism offers excellent sensitivity and repeatability across a wide pressure range, typically from vacuum to several thousand psi.
Pressure spring tubes find extensive applications in various industrial and scientific instruments, including: