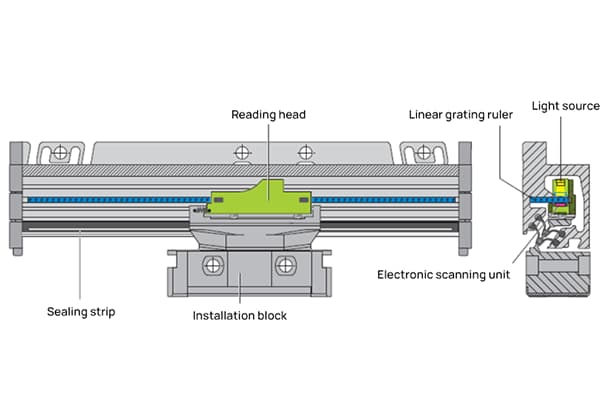
How do manufacturing processes blend heat and pressure to create superior materials? Thermomechanical treatment, a method combining deformation and heat treatment, enhances mechanical properties and saves energy. This article explores 36 types of thermomechanical processes, highlighting techniques such as hot-forging quenching and rolling quenching, and their applications in improving the strength and durability of various steel products. From boring cutters to reinforced bars, discover how these processes transform material performance and efficiency.
The process of deformation heat treatment is commonly referred to as “thermo-mechanical processing.”
In the field of machinery manufacturing, the combination of pressure processing techniques (such as rolling, forging, and rolling) and heat treatment can result in both linear and heat treatment reinforcement, leading to comprehensive mechanical properties that cannot be achieved through a single reinforcement method.
This combined strengthening process is referred to as thermomechanical treatment.
In addition to providing exceptional mechanical properties, thermomechanical treatment also eliminates the need for high-temperature heating during heat treatment, resulting in significant energy savings, reduced use of heating equipment and workshop space, and reduced occurrences of heat treatment defects such as material oxidation, decarburization, and distortion.
Therefore, the thermomechanical treatment process not only provides excellent strengthening effects, but also offers significant economic benefits.
For reference, the following 36 examples of thermomechanical treatment methods are listed.
A boring machine with a boring cutter body diameter of 4mm, head diameter of 6mm, and total length of 40mm is immediately quenched after forging and promptly tempered. This results in a cutting life increase of over 30% compared to conventional treatments.
A self-use turning tool made of M2 steel from a domestic machine tool electrical factory is quenched in oil immediately after forging and tempered at 550°C. This results in a service life that is more than one time greater than that of market lathe tools.
The self-use 9341 steel 12-square turning tool of Jialong Company is oil-cooled after free forging, resulting in a relatively long service life.
The hot-forging quenching process for a 355mm x 98mm x 33mm crusher hammer made of 65Mn steel is as follows:
The initial forging temperature is 1050°C, and the final forging temperature ranges from 840°C to 860°C. After the final forging, the hammer should be allowed to cool in air for 2 to 3 seconds, then quenched in flowing tap water. It should then be tempered at a temperature range of 180°C to 200°C, resulting in a surface hardness of 50 to 55 HRC within 10mm of the surface.
This hot-forging quenching process increases the service life of the hammer by more than 50% compared to conventional heat treatment.
A 40Cr steel socket wrench from a domestic hardware tool factory uses forging quenching instead of the traditional salt bath quenching. This method is not only energy-efficient and environmentally friendly, but also produces high-quality results.
The 55MnSi steel chisel is forged using a 2500N air hammer and a specialized die. The optimal temperature for deformation is between 920-950℃, with a deformation rate of approximately 75%. The final forging temperature is around 900℃.
To maintain the optimal hardness and toughness, the workpiece should be quickly quenched in water and cooled in oil within 30 seconds of deformation (based on the color of the workpiece’s surface). The chisel should then be tempered at a temperature range of 220-270℃.
After undergoing thermomechanical treatment, the chisel has improved hardness and toughness, resulting in a longer service life.
The 230mm x 120mm CrMn steel blank, weighing approximately 40kg, is forged into 90mm x 90mm x 600mm square bars. Blanking is then performed according to the ring gauge size.
The blank will be heated to a temperature of 1050 to 1150 ℃ with proper insulation. It will then undergo rapid upsetting-extrusion forming in the high temperature deformation area.
The shape variation will be between 35% and 40%, with a final forging temperature of 920 to 900 ℃.
Immediately after forging, the square bar will be cooled in oil at a temperature of 40 to 70 ℃ for 40 to 60 seconds.
After air cooling to approximately 100 ℃, the square bar will be tempered.
The surface hardness of the ring gauge must be ≥62HRC.
The initial forging temperature ranges from 1070 to 1150°C, while the final forging temperature is set at 850°C. The deformation variable ranges from 35% to 75%. The tempering temperature can range from 200 to 350°C.
Compared to heating and quenching in a salt bath fire box furnace, strength has increased by approximately 30%, and wear resistance has increased by 26% to 30%.
The deformation temperature ranges from 930 to 970°C, with a deformation variable of 30%. Cooling is done using oil, and the tempering temperature ranges from 150 to 180°C.
Compared to conventional heat treatment methods, this process results in a nearly 20% increase in strength and a 23% increase in contact fatigue life.
The initial forging temperature is between 1150 and 1180℃ with tread forging, and the deformation time is between 13 and 17 seconds, with a deformation rate of approximately 40%.
Afterwards, the workpiece is immediately trimmed on a 2150N crank press, followed by immediate quenching (at which time the temperature of the workpiece is between 900 and 950℃), and then tempered at 650℃.
When working with 45Mn2 steel balls with a diameter of 70 to 100mm, the initial forging temperature should be around 1200°C. The final forging temperature should be maintained between 1000°C and 1050°C.
The appropriate pre-cooling time after water quenching can be selected based on the steel ball specifications. Tempering the steel balls at a temperature between 150°C and 180°C will result in a surface hardness of at least 57 HRC, with a hardened layer depth of more than 20mm and a hardness greater than 50 HRC. This meets the requirements for large-specification steel balls.
The intermediate frequency induction heating temperature is between 1100°C to 1200°C. During the roll forging process, from the start of deformation to 20 seconds before quenching, the deformation of the plowshare’s various parts ranges from 56% to 83%. After deformation, the quenching heat density is between 1.30g/cm3 to 1.35g/cm3 in a CaCl2 aqueous solution.
After quenching, the plowshare is tempered at a temperature of 460°C to 480°C for 3 hours, resulting in a hardness range of 40 to 45 HRC.
Compared to the traditional heat treatment process for plowshares, the number of heating cycles has been reduced from 4 to 5 times to just two times, leading to an increase in production efficiency of about 4 times. The product quality meets first-class requirements, resulting in significant economic benefits.
For the 40Cr steel steering knuckle with a diameter of 60mm, it is forged by heating it to a temperature range of 1150 to 1200℃. The final forging temperature is then reduced to 900 to 850℃ and oil cooling is conducted. The knuckle is tempered at a temperature of 600℃ for 2 hours.
The use of waste heat from the forging process for quenching not only saves energy and reduces costs, but also significantly improves the organizational structure and material performance, particularly in terms of impact toughness, which is crucial for automotive safety.
The overall dimensions of the die are 70mm x 20mm x 10mm.
There are 20 small holes on the 20mm width plane, with diameters of 1.5mm, 2.5mm, and 3mm. These holes require heat treatment with a hole spacing tolerance of ±0.006mm, flatness less than 0.01mm, and hardness between 56 and 60HRC.
Due to the severe segregation of eutectic carbides in Cr12MoV steel, there is a significant risk of cracking after billet rolling. The material is still distributed in strips along the rolling direction, with the core being distributed in mesh, block, and piles, which become stress concentrations and sources of cracks. This leads to material anisotropy and increased heat treatment distortion.
Forging heat deformation is a better solution for addressing these problems.
The specific process is as follows:
The initial forging temperature range is 1050°C to 1160°C, with a final forging temperature range of 850°C to 950°C.
The material undergoes oil cooling when hot, followed by two tempering processes at 780°C for 3 hours each.
The final metallographic structure consists of martensite, lower bainite, dispersed carbide powder, and small amounts of residual austenite.
The specific volume is similar to that of thermally tempered sorbite.
Micro-deformation does not require straightening after heat treatment, and all distortions meet technical requirements with a hardness range of 58 to 60 HRC and a qualified rate of 99.99%.
This heat treatment process results in high heat resistance, thermal hardness, wear resistance, and a long service life for the die.
Examples of quenching with forging waste heat and high temperature tempering include hexagonal drawing dies, deep drawing dies, and cold punching dies, among others, but these are not mentioned here.
Miscellaneous metal wares and tools, such as wrenches, screwdrivers, pliers, and scissors, were among the first to be hardened through the residual heat generated during forging. This could be considered the first prototype of thermomechanical treatment.
The tool parts were heated and then quenched in a coke furnace, where the fire color was observed, a process known as line forging. Some required multiple heating cycles to reach the desired size, while the final forging step after forming did not require air-cooling.
The appropriate coolant should be selected based on the material and then applied to the side of the furnace or tempered using its residual heat. A special tempering furnace is rarely used.
After undergoing free forging, woodworking tools such as planers, axes, and chisels are typically quenched using residual heat. This method is cost-effective, as it saves on electricity and time and is also highly efficient in terms of production.
In some rural market towns, coke ovens are still utilized.
Agricultural machinery cooled by the residual heat from forging includes sickles, shovels, rakes, crusher hammers, as well as kitchen utensils such as spoons, spatulas, and knives.
The steel tends to develop chain-like carbides after slow cooling during forging, resulting in die brittle fracture, cracking, or thermal cracking failure.
Heating at normal temperatures can dissolve M6C.
When air-cooled at a speed greater than 15 ℃/min, which exceeds the critical cooling speed for the formation of chain carbides, it eliminates chain carbides and anneals the steel through spheroid annealing to achieve uniform fine distribution of carbides.
The recommended normalizing temperature is 1130 ℃. This modification results in a reduction in the forging normalizing impact toughness from 26J/cm2 to 23J/cm2 and an increase in service life from 1500 pieces to 2000 pieces.
The process of high temperature deformation normalizing involves heating the workpiece to its final forging temperature of approximately 850°C and then allowing it to cool in the air. This not only enhances the strength of the steel but also significantly improves its impact toughness, wear resistance, fatigue resistance, and reduces its brittle transition temperature.
The 20CrMnTi steel forging blank has dimensions of 80mm x 80mm x 40mm.
After forging, the workpiece is cooled by air, and the cooling speed is carefully controlled to enhance its mechanical properties and make it easier to cut.
Some domestic machinery companies producing 20CrMnTi steel automotive gears use the waste heat generated during forging to perform normalizing. This process can save over 300kW·h of electricity per ton of gears produced.
Some domestic units immediately place the high-speed steel into Ac1 (20-30°C) for 2-3 hours after forging, allowing the furnace to cool to 550°C and then air-cooling. This simplifies the process, shortens the production cycle, and saves 70-90% in electricity costs, reducing production costs and improving working conditions. Additionally, this process improves the quality of the forgings and facilitates mechanized operations.
For high-speed steel workpieces processed through rolling, die forging, and isothermal processing, there is no need to follow the traditional annealing process. This example can be used as reference.
The dimensions of the die are 250mm x 200mm x 42mm. The starting forging temperature is between 1150-1100°C and the final forging temperature is between 900-850°C.
The annealing process involves heating the die to 800-820°C for 4-6 hours, then allowing the furnace to cool to 500°C using air cooling.
In the wood processing industry, some rotary and planing knives are manufactured using the flanging method. The blade of these knives is made from an alloy tool steel, such as 5Cr8W2MoVSi, while the body or back is made from 45 Q235A steel. The body is heated to the forging temperature of the blade steel and then the two are welded together using a rolling mill.
This process is known as solid phase welding, and the blade is rolled to the desired size before being controlled to the final rolling temperature and then promptly quenched and cooled.
The blades produced through this method are of high quality, with high hardness and long life, and offer the added benefits of saving time and electricity during the manufacturing process.
Rolling hot quenching is a heat treatment process that uses the residual heat generated from rolling various profiles to quench them. This process yields the same strengthening effect as forging hot quenching.
For example, M2 steel can be rolled at a temperature of 1220°C (250 rolling mill, 50r/min) to the desired size and then directly quenched, resulting in a hardness of 65HRC or higher. This results in a longer cutting life for lathe tools compared to salt bath quenching.
The author has successfully executed a thermomechanical treatment process using twist drills from a domestic tool company for machine use.
The high-frequency heating device was utilized to conduct four-roller hot rolling.
The austenitizing temperature was set between 950°C and 1000°C, and the deformation temperature was between 880°C and 950°C, with a deformation rate of approximately 30%. The quenching cycle was performed using a two-nitrate aqueous solution, with water temperature kept below 70°C.
The resulting hardness after quenching was ≥54 HRC, and after tempering at a temperature of 240°C to 260°C for 1 hour, the hardness was ≥50 HRC, which meets the technical requirements and exceeds the deformation requirements for more than 95%.
20MnSi steel reinforced bars require hot-rolled supply and must meet the performance requirements of having a tensile strength of ≥ 510MPa, a flexural strength of ≥ 335MPa, and an elongation of ≥ 16%.
A 60mm x 60mm billet is rolled into a 16mm diameter reinforced bar. The initial rolling temperature ranges from 1100 to 1200°C and results in a rolling shape reduction of around 93%. The final rolling temperature is between 950 to 900°C, which is the temperature for low-carbon martensite quenching of the steel.
After rolling, the bar is cooled with water within 1 to 1.26 seconds. It then undergoes self-tempering at temperatures between 550 to 600°C.
The reinforced bar that has undergone the above rolling, quenching, and tempering process boasts mechanical properties that exceed those specified in GB1499 and also surpass the mechanical properties specified in the British BS4449 standard.
The extrusion deformation temperature ranges from 1100 to 1200℃, and the tempering temperature is between 570 to 580℃.
The hardness of the material is between 300 to 335HBW, with a tensile strength of ≥ 1068MPa, flexural strength of ≥ 960MPa, and an elongation of ≥ 14.5%, which satisfies the standards set by the Ministry of Standards.
Experience shows that for large extrusion waste heat quenching workpieces such as joints, it is crucial to carefully select the deformation temperature, the time elapsed before quenching after deformation, the quenching medium, the cooling time of the workpiece in the quenching medium, and the tempering temperature, among other process parameters.
The purpose of the 840°C x 2h oil cooling and 200°C x 2h tempering process is to achieve double refinement of the tissue.
Then, during the superplastic deformation process at 800°C, the deformation rate is 2.5 x 10s and the tensile deformation variable is 250%. After deformation, cooling in oil is performed.
The results of the superplastic deformation test on the steel, including the bending strength, multi-stroke life, and hardness indicators, showed that the flexural strength was 28% higher than with conventional treatment. The multi-stroke life increased by 38.6%, and the hardness was ≥ 60 HRC, equivalent to that achieved through conventional quenching.
The bending strength of H11 steel is 1852 MPa, and after undergoing two tempering cycles at 482°C in conventional quenching, its elongation rate is 12.5%.
By conducting a low-temperature deformation quenching and two temperings at 482°C, followed by 2% deformation aging at around 316°C and a final tempering at 482°C, the flexural strength of the steel increases to 2548 MPa, an increase of 37.5%, while its elongation rate remains unchanged.
This compound thermomechanical treatment is a process in which high-temperature deformation quenching is followed by a small amount of deformation and tempering at a specific temperature.
Conducting martensite deformation aging after high-temperature deformation quenching can result in the steel obtaining much higher strength properties than any other heat treatment.
For instance, the mechanical properties of 50CrVA after conventional quenching and 200°C tempering are a tensile strength of 2119 MPa, a flexural strength of 1497 MPa, and a 41.7% reduction in section.
After undergoing high-temperature deformation quenching, 200°C tempering, 3% deformation, and 200°C tempering, the mechanical properties of 50CrVA are a tensile strength of 2597 MPa and a flexural strength of 2254 MPa.
This composite thermomechanical heat treatment, combining high-temperature deformation quenching and martensitic deformation aging, has increased the tensile strength and flexural strength of 50CrVA steel by 22.6% and 50.7%, respectively.
The Jialong Company heats and quenches mechanical blades, such as planing knives and rotary knives with lengths greater than 2 meters, in a protective atmosphere furnace at a temperature of approximately 500℃.
After the workpiece has cooled to around 200℃, it is rolled back and forth on a roller press several times, utilizing the principle of phase change superplasticity. This process allows for immediate adjustment of straightness to ≤0.30mm after bending 10-15mm.
Not only does this deformation reinforcement straighten a previously bent insert, but it also creates residual compressive stress of approximately 5mm depth on the rolled surface. This helps to enhance the tool’s lifespan.
The process involves carburizing after cold deforming the workpiece, as the cold deformation creates various structural defects that can hasten the carburizing process.
For instance, after cold heading, the deformation of 20CrNiMo is 25%. If the workpiece is gas carburized at a temperature of 930-950°C for 2 hours, the depth of the carburizing layer will reach 0.84mm. If the deformation increases to 50%, the depth of the coating will reach 0.88mm. The greater the deformation, the deeper the penetration layer.
The process is a composite heat treatment in which the workpiece undergoes nitriding after being cold deformed at room temperature.
Cold deformation nitriding is distinct from cold deformation carburizing.
Cold deformation decreases the rate of nitrogen penetration and decreases the thickness of the diffusion layer, and this trend becomes more pronounced as the level of deformation increases.
This phenomenon may be caused by nitrogen atoms impeding the diffusion of other nitrogen atoms by pinning dislocation sites or trapping dislocated nitrogen atoms.
However, cold deformation nitriding can increase the toughness of pure iron.
The nitriding temperature and duration depends on the type of steel, for example, 38CrMoAl steel and 20 steel require temperatures of 650°C and 550°C, respectively.
This is a combined heat treatment in which the workpiece undergoes room temperature deformation followed by boron infiltration.
For instance, 20 steel workpieces are rolled and deformed in a greenhouse, then subjected to a 900°C holding period and solid boron infiltration with varying heating rates.
Tests have demonstrated that cold deformation significantly increases the depth of the boron infiltration layer.
The optimal level of deformation for maximum penetration depth varies based on the heating rate and holding time during the boron infiltration process.
This phenomenon is caused by the cold deformation of the steel’s structure, which accelerates the boron atomic adsorption process on the steel surface.
Cold Deformation Carbonitriding is a composite heat treatment process where medium temperature carbonitriding is carried out after a room temperature deformation process.
The pre-treatment step of cold deformation has a significant impact on the carbonitriding process of steel, as it increases the C and N content on the surface and enhances the thickness of the penetration layer.
For instance, when the cold-rolled deformation of 20CrMnTi steel is 15%, the thickness of the carbon and nitrogen co-infiltration after 860℃×2h and 860℃×4h processes are 0.65mm and 0.80mm, respectively.
Deformation at room temperature affects not only the diffusion process of interstitial atoms in steel, but also the penetration process of substitutional atoms.
As an illustration, the cold deformation of 16Mn steel was studied to examine the effect on the solid infiltration process of titanium. The results showed that the best temperature for titanium infiltration was 900 to 950℃, with a deformation of 30%.
Furthermore, as the temperature of titanium carburizing increases, the holding time also increases, leading to a thicker penetration layer.
The thermomechanical heat treatment process involves heating the workpiece blank to the initial forging temperature for forging, followed by carburizing in a carburizing furnace, and finally, direct quenching.
The forging heat-carburizing-quenching method conserves electrical energy that would otherwise be required for heating the workpiece during carburizing and increases the speed of carburizing. This results in improved surface hardness, wear resistance, and makes it suitable for medium modulus gears and other carburized workpieces.
Another form of combined carburizing and thermomechanical treatment is called carburizing-forging-quenching, which involves carburizing followed by hot-die forging and quenching.
This process can significantly increase the thickness of the effective hardened layer on the workpiece, increase surface compressive stress, improve resistance to breakage, and extend the product life.
The hardness of a 9SiCr steel circular screwing die after heat treatment is typically between 62 and 65 HRC. The conventional heat treatment process involves heating in a salt bath at a temperature of 860 to 880℃, followed by quenching and tempering at 150 to 180℃.
To improve the tool hardness and wear resistance, surface chemical heat treatment can be used. However, this process requires a temperature of at least 400℃, which is not suitable for 9SiCr steel tools. Nitriding, on the other hand, can provide a solution to this problem.
The nitriding process involves heating the tool in an LD 60kW ion nitriding furnace, followed by a 100kW medium temperature salt bath furnace, oil cooling, cold treatment, and finally tempering at 150 to 180°C.
Testing has shown that the hardness at a depth of 0.10 to 0.80mm is greater than 927HV5, with a peak hardness of 974 to 986HV5. The hardness at a depth of 0.20 to 0.60mm is ≥857HV5, which improves the anti-tempering properties of the hardened area and extends the lifespan of the material.
The thermomechanical treatment process is extensively utilized.
From a material perspective, it is appropriate for a wide range of metal materials including various carbon steels, alloy steels, alloy structural steels, and nickel-based alloys.
In terms of processing methods, it can combine the benefits of both to fulfill specific requirements for strength and toughness, significantly enhancing the quality and longevity of deformed components.
The future outlook for thermomechanical treatment is positive.