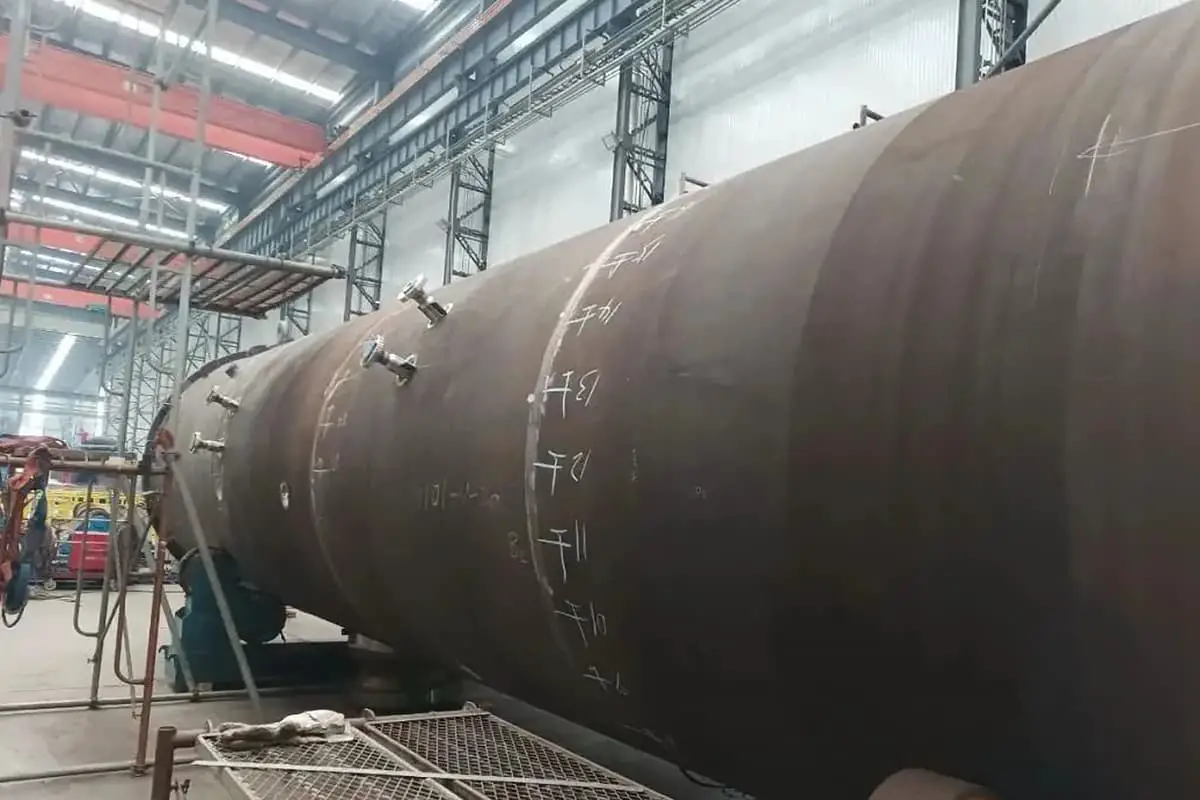
How can we join aluminum and steel, two metals with vastly different properties, without compromising their structural integrity? This article delves into advanced laser welding techniques to address this challenge, exploring methods that minimize brittle intermetallic compounds and enhance joint strength. Discover the latest research and practical insights into creating strong, reliable aluminum-steel connections, crucial for industries like automotive and aerospace. Learn how innovations in welding technology are paving the way for more efficient, lightweight, and durable metal structures.
Connecting aluminum and steel can achieve lightweight automotive parts. However, intermetallic compound cracking caused by welding these dissimilar metals can significantly impact joint performance.
This post introduces the main welding methods for laser welding of aluminum and steel and explains the research status in recent years.
Galvanized steel is a steel substrate coated with layers of zinc of different thicknesses. The process of zinc plating is categorized into electroplating and hot plating.
The zinc layer not only provides physical shielding but also offers electrochemical protection to the steel substrate.
Due to its excellent corrosion resistance, galvanized steel is widely used in various industries such as transportation, electric power, construction, heating facilities, instruments, and furniture.
In particular, the application of ordinary galvanized steel, high-strength galvanized steel, and ultra-high-strength galvanized steel in the automobile industry improves the corrosion resistance and extends the service life of the body and other parts.
However, the presence of a zinc layer makes melting welding of galvanized steel plates challenging. This is because zinc’s melting point is 420 ℃, its boiling point is 908 ℃, while the base steel’s melting point is 1300 ℃ and its boiling point is 2861 ℃.
During the melting and welding process, zinc tends to evaporate and oxidize extensively, leading to defects such as pores, incomplete fusion, and cracks.
Aluminum and its alloys are known for their lightweight properties (with a density of 2.7g/cm3), high specific strength, excellent electrical and heat conductivity, corrosion resistance, and the ability to retain good mechanical properties even at low temperatures.
In today’s world, energy, safety, and environmental protection have become crucial issues. With the growing concern about environmental pollution and the energy crisis, achieving vehicle lightweight has become a global focus.
Consequently, extensive research has been conducted on aluminum/magnesium, aluminum/titanium, titanium/aluminum, and aluminum/steel composite structures worldwide.
Aluminum/steel composite structures make optimal use of the advantages of both materials to reduce weight and pollution, and they have found wide-ranging applications in the automotive, aviation, and shipbuilding industries.
The application of aluminum-steel connections in the automobile door panel is depicted in the figure below.
Connecting aluminum and steel is extremely challenging due to their significant differences in thermophysical properties.
Iron has a melting point of 1538 ℃ and a density of 7870 kg/m3, while aluminum has a melting point of 660 ℃ and a density of 2700 kg/m3.
During the welding process of aluminum and steel, the aluminum will float on the steel when the latter is fully melted, making it difficult to create a weld. Additionally, aluminum has a linear expansion coefficient almost twice that of iron, leading to high thermal stress and an increased risk of cracking during welding.
The Fe-Al binary phase diagram shows that iron and aluminum can form solid solutions, intermetallic compounds, and eutectics.
However, the solid solubility limit of iron in aluminum is minimal.
At temperatures ranging from 225 to 600℃, the solid solubility of Fe in Al ranges from 0.01% to 0.022%. At the eutectic temperature of 652℃, the solubility of Fe in Al is 0.53%. Numerous studies have shown that Fe and Al can combine to form intermetallic compounds, such as Fe3Al, FeAl, FeAl2, Fe2Al5, and FeAl3, which can cause brittle structures in the weld.
However, not all intermetallic compounds develop during the welding process, according to thermodynamic analysis of Fe-Al. Based on Gibbs free energy calculations, only Fe2Al5 and FeAl3 are the last stable brittle structures.
The presence of brittle structures can reduce the mechanical properties of welded joints and lead to crack formation easily.
Aluminum and steel fusion welding can be challenging due to the limited solubility of Fe in aluminum, resulting in difficulty in obtaining a sound joint.
To prevent the formation of brittle intermetallic compounds between steel and aluminum, the steel surface must be coated with metals compatible with both aluminum and iron, such as Ni, Zn, Ag, and Cu.
This coating will help avoid or minimize the formation or growth of Fe-Al intermetallic compounds.
Different coating metals have different effects on the reaction between steel and aluminum interfaces, and the weldability of dissimilar metals can also vary.
In addition to improving the weldability of steel and aluminum, the selection of appropriate filler materials and flux can also enhance the weldability of dissimilar metals and expand their application in various industries.
The main characteristic of laser deep penetration welding is its ability to create deep penetration holes.
This process involves high power density laser irradiating the metal surface, causing the surface metal to reach its boiling point and rapidly melt and vaporize.
The resulting evaporation of the metal generates air pressure, causing the surface to become concave and form small holes.
These small holes increase the energy absorption of the laser.
The heat generated during this process comes from the melting of the metal around the small hole, as well as the liquid flow outside of the hole, the surface tension of the inner wall of the hole, and the continuous steam pressure in the inner cavity of the hole.
As the laser beam continues to enter the small hole, the material outside of the hole continuously melts and flows.
With the movement of the laser beam, the small hole remains in dynamic stability.
As the light beam moves, the molten metal surrounding the small hole moves with it, continuously filling the small hole until it cools and solidifies to form a weld.
In laser deep penetration welding for connecting aluminum steel, the most commonly used joint form is the steel upper and aluminum lower.
The laser beam is directed at the steel surface, causing both the steel and aluminum plates to melt and form a welding hole.
Gsierra et al. conducted a study on the laser deep penetration welding of steel with the upper aluminum lap and lower lap joint configuration.
The research findings suggest that controlling the weld penetration to below 500 μm can reduce the formation of Fe-Al intermetallic compounds and minimize weld brittleness.
By controlling the weld penetration to below 500 μm, the joint strength can reach 250 MPa.
However, there may be some intermetallic compounds and white molten bands, which are formed due to aluminum-rich compounds, present in the weld.
When the penetration depth is less than 500 μm, the junction between the weld and aluminum alloy is the point of failure for the joint.
With an increase in penetration depth, the joint failure position shifts, and the joint strength significantly decreases. Kouadri David et al. conducted a study on the microstructure and properties of laser deep penetration welding and laser thermal conductivity welding of galvanized steel and aluminum alloy.
By limiting the weld penetration to 600μm, the strength of the laser deep penetration welded joint reached 140MPa. The study pointed out that the penetration along the steel thickness direction had a significant impact on the joint strength.
Similarly, Katsuyama et al. demonstrated that the penetration depth of steel in aluminum was the key factor affecting joint performance.
Toryamany et al. examined the Nd: YAG pulsed laser welding of low carbon steel / 5754 aluminum alloy using laser deep penetration welding overlapping structure in the experiment.
A study was conducted on the effects of laser power, pulse width, and lap factor on the formation of metal compounds. The results indicate that the amount of intermetallic compounds increases with an increase in laser peak power (at constant pulse energy), pulse width (at constant peak power), and lap factor (at constant pulse energy and peak power).
Jinyang et al. investigated the relationship between penetration and weld formation in laser deep penetration welding of pure aluminum/stainless steel. The results show that when the penetration is large (354μm), a Fe-Al intermetallic compound with microcracks is formed at the aluminum/fusion zone interface, which is rich in aluminum. The joint strength is (27.2 ± 1.7) MPa. The fracture occurs in three forms: shear brittle fracture, cleavage brittle fracture, and mixed fracture.
In contrast, when the penetration is small (108), the interface of the Al/Fe fusion zone is crack-free intermetallic compound, and the joint strength is (46.2 ± 1.9) MPa. Only one form of fracture, cleavage brittle fracture along the weld, is observed.
The advantages of laser deep penetration welding include high utilization rate of laser energy and efficient welding.
The keyhole plays a crucial role in weld penetration and width, and it is a vital factor in the laser deep penetration welding process.
However, the plasma and deep penetration holes formed during welding can make the process unstable and difficult to control.
Furthermore, the entrance of gas into small holes and the formation of solidification pores are common occurrences during the laser deep penetration welding process.
The steam pressure generated by metal steam can cause surface depression and unsightly welds due to metal shrinkage during solidification.
When a laser beam irradiates the surface of a material, some of the laser is reflected while the rest is absorbed by the material. The absorbed laser energy is converted into heat energy, which causes the material to melt.
The heat on the material surface then propagates through the material via heat conduction, eventually welding the two materials together.
Laser thermal conduction welding is an important welding process in laser welding, particularly for thin parts. Heat conduction dominates the process of heat propagation in this welding mode, with radiation and convection playing a minor role that can be ignored.
Additionally, the weld pool in laser thermal conduction welding is small, so the impact of thermophysical parameters with temperature and state on the welding thermal process and the release of phase change latent heat in the weld pool can be ignored.
MECO and other researchers have used laser heat conduction welding to join a 2mm thick steel plate and a 6mm thick aluminum plate in an overlapping form, with steel on top and aluminum on the bottom. When the laser irradiates the surface of the steel plate, the transmitted heat melts the aluminum alloy at its melting point.
The resulting thickness of the intermetallic compound was 4-20μm, with the maximum microhardness of Fe2Al5 being 1145HV.
Laser arc hybrid welding technology, as shown in the experimental schematic diagram in Fig. 2, is a new and efficient welding method developed in the 1970s.
Laser high energy density allows for deeper penetration, but the bridging of the gap is poor, and the assembly accuracy must be high.
The arc’s heating range is wide, which allows for a wider weld to be obtained, but the bridging of the arc to the gap is good.
By utilizing their respective characteristics, laser arc hybrid welding technology can obtain a wide top and large penetration weld.
The plasma produced by the laser can stabilize the arc, making the hybrid welding process adaptable and efficient.
Honggang Donga et al. invented a method for connecting dissimilar metals using large spot laser and arc composite heat sources.
The patent focuses on laser arc hybrid heat source welding with a small spot, which cannot be applied to the connection between steel and aluminum, steel and copper, and other dissimilar metals.
In this method, the laser has an obvious stabilizing effect on the arc, which is used to melt the filler metal and low melting point base metal.
The large spot laser can accurately control heat input.
Tensile property tests on 5A02 aluminum alloy and galvanized steel joints obtained by this method show that the failure position of the sample occurs in the welding heat affected zone on one side of the aluminum alloy base metal, rather than the brazing connection zone. The joint strength can reach 153.1 MPa. Qin and others adopt laser MIG composite welding of galvanized steel/aluminum alloy and AlSi5 welding wire.
Experimental results show that the brazing interface produces 2 to 4 μm, and the phase groups are FeAl2, Fe3Al5, and Fe4Al13. The maximum tensile strength of the joint is 247.3 MPa.
Wangshujun et al. also used laser MIG hybrid welding technology and experimented with three different solders: AlSi5, AlSi12, and AlMg5 to study the effects of intermetallic compounds, weld microstructure, and formation after the addition of Si and Mg.
The results show that increasing Si content can refine the grains in the melting zone and increase the microhardness of the melting zone. The microhardness of Al-Si solder in the melting zone is greater than that of Al-Mg solder.
The average thickness of the intermetallic compound layer for AlSi12, AlSi5, and AlMg5 solders is 0.90 μm, 1.49 μm, and 2.64 μm, respectively.
It has been concluded that the presence of Si in the solders inhibits the diffusion of Fe and reduces the formation of intermetallic compounds.
XRD analysis of the intermediate layer reveals that the intermetallic compound layers corresponding to AlSi5 and AlSi12 consist of Fe2Al5, Fe4Al13, and Al0.5Fe3Si0.5 phases, whereas those corresponding to AlMg5 consist of FeAl2, Fe2Al5, and Fe4Al13 phases.
The joint strength of AlMg5, AlSi5, and AlSi12 solders are 178.9 MPa, 172.43 MPa, and 144 MPa, respectively.
It has been concluded that an increase in Si content is detrimental to joint strength, whereas the addition of Mg enhances joint strength. However, it has not been explained how Si and Mg affect joint strength.
Thomy et al. conducted a study on the interaction between laser and plasma arc during laser arc hybrid welding and developed a coaxial laser arc hybrid welding head.
Brazing involves using solder with a lower melting point than the base metal. By heating to a temperature higher than the melting point of the solder and lower than the melting point of the base metal, the solder melts while the base metal remains solid.
The liquid solder then wets the base metal and, under the capillary action of the brazing gap, automatically fills any gaps in the base metal and diffuses with it to create a strong joint.
Fusion brazing combines the characteristics of brazing and fusion welding, making it ideal for connecting dissimilar materials with large differences in physical properties.
When steel and aluminum are fused-brazed, the steel remains solid while the aluminum and solder melt. The resulting brazing connection is formed on the steel/solder side, while the fusion welding occurs on the solder/aluminum side.
The core principle of fusion brazing for steel and aluminum is to combine molten aluminum and solder with solid steel through an interface reaction.
In this method, solder may or may not be added for welding purposes.
Peyre et al. conducted a study on the bonding of galvanized steel and aluminum alloy using laser fusion brazing without solder.
Their findings indicate that a 2 to 20 μm thick interface layer forms along the steel-aluminum interface.
The layer primarily consists of the Fe2Al5 phase, with a hardness of up to 1200HV. This leads to cracks in the joint, causing a significant reduction in the mechanical properties of the joint.
The evaporation of zinc produces pores, but a 10μm-thick zinc coating promotes the wetting and spreading of aluminum on steel.
Tensile tests indicate that coating flux on the surface of galvanized steel can inhibit zinc evaporation. Non-galvanized steel, however, exhibits low mechanical resistance.
To control the formation of Fe-Al intermetallic compounds and enhance joint performance, some researchers have used solder during laser brazing to alter the joint’s chemical composition.
Sierra et al. utilized 4047 (Al-12Si) solder in the aluminum/steel laser fusion brazing connection, resulting in a continuous joint without significant macro defects and a thin Fe Al-Si intermetallic compound layer at the steel/weld interface.
The effect of Si on the growth of Fe-Al intermetallic compounds was investigated by using Al-12Si solder. The proposed mechanism suggests that Si reduces the melting temperature of aluminum, influences the viscosity and surface tension of molten aluminum, and subsequently affects the weld wetting angle and width.
Additionally, some scholars have added Mg, Cu, and other elements to the solder to explore the effect of solder alloying on the weld’s microstructure and properties.
Dharmendra et al. conducted a fusion brazing test on a lap joint made of DP600 galvanized steel and AA6016 aluminum alloy using continuous pulse Nd: YAG laser.
They used Zn-Al welding wire containing 85% Zn and 15% Al in the test, and varied the laser power, welding speed, and wire feeding speed.
The researchers found that the thickness of the reaction layer was between 3 and 23μm.
When the heat input was between 60 and 110J/mm, the joint’s tensile strength reached 220MPa, with the fracture position located far away from the weld and close to the aluminum alloy side.
At welding speeds of 0.5 and 0.8m/min, the corresponding intermetallic compound thicknesses were 8 and 12μm, respectively.
Tensile testing showed that the joint had the highest mechanical resistance when the intermetallic compound thickness was between 8 and 12μm.
If the thickness was less than 8μm, mechanical resistance increased as the intermetallic layer increased, while if it was greater than 12μm, mechanical resistance decreased.
It has been explained that when the compound layer is thin, the crack initiates along the brittle intermetallic compound layer, resulting in very low fracture strength.
On the other hand, when the compound layer is thick, the mechanical resistance is also low due to the brittleness of the layer relative to other areas.
Laukant et al. conducted a laser fusion brazing test using ZnAl2 solder on aluminum/steel. The results showed that intermetallic compound layers of about 5μm FeAl were produced, and the joint shear force reached up to 9 KN.
Similarly, Rajashekhara Shabadi et al. conducted a laser fusion brazing test using ZnAl30 solder on AA6016 and low carbon galvanized steel. The intermetallic compound formed in the test results is mainly Fe2Al5Znx, which may also contain ZnFeAl3, with a thickness of about 10μm.
Recently, some scholars have conducted laser fusion brazing experiments on aluminized high-strength steel and aluminum alloy.
For instance, Windmann et al. performed a laser fusion brazing test using AlSi3Mn solder and discovered the formation of the Al8Fe2Si phase at the AlSi3Mn/Mn22B5 interface.
Moreover, the thickness of the intermetallic compound created at the Mn22B5/AlSi3Mn interface is 2 to 7μm, and the joint’s shear strength is 21 to 74MPa.
By preheating the steel surface before welding, the joint strength can increase to 210 to 230MPa.
Recent research has shown that both Al-Si solder and Zn-Al solder inevitably create intermetallic compounds.
Nevertheless, the growth sequence of Fe Al-Si intermetallic compounds and the identification of the Zn-Al solder reaction phase require further investigation.
Laser fusion welding is a promising technology for connecting steel and aluminum.
Laser arc hybrid welding is mainly used for welding thicker plates due to its high welding efficiency.
Laser fusion brazing holds great potential for application in lightweight automobile production.
The laser fusion brazing process was used to connect thin dissimilar metals made of aluminum and steel, utilizing Al-Si and Zn-Al solders.
However, there remain several challenges in the laser welding of galvanized steel/aluminum alloys for automobile manufacturing. For instance, the low absorptivity of the material to laser energy results in plasma generation, which can impact the welding process’s stability.
Additionally, the process of connecting aluminum and steel can produce brittle Fe-Al intermetallic compounds. Furthermore, the molten solder’s metallurgical compatibility with the aluminum alloy base metal and its wettability to the base metal steel need to be considered.
Finally, it is crucial to control and prevent welding defects such as pores, cracks, incomplete fusion, and slag inclusion.