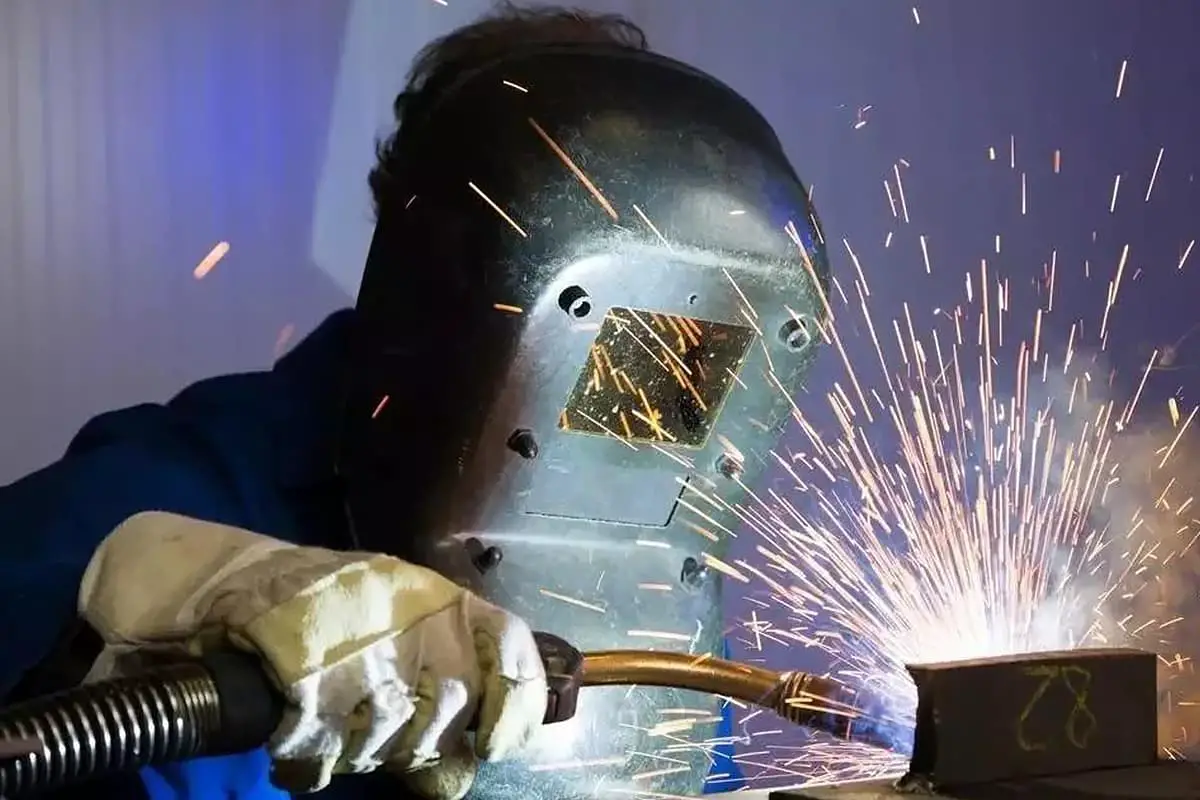
Have you ever wondered why welded structures sometimes fail despite their robust appearance? This article dives into the hidden challenges of welding, exploring how uneven heating and cooling can lead to stress, distortion, and even structural failure. By the end, you’ll understand the key factors affecting welding quality and how to mitigate these issues.
Welded structures, while widely used in industry, often present inherent challenges primarily associated with the welded joints. These challenges can be categorized into several key areas:
1. Residual Stress and Distortion:
The welding process induces localized, intense heating followed by rapid cooling, resulting in non-uniform thermal expansion and contraction. This thermal cycling leads to residual stresses and distortion in the welded structure. These issues not only compromise dimensional accuracy and aesthetic quality but also complicate post-welding operations. In severe cases, the overall structural integrity and load-bearing capacity may be significantly impaired.
2. Microstructural Changes:
Welded joints undergo three distinct phases during formation: melting, solidification, and heat-affected zone (HAZ) development. Each phase alters the material’s microstructure, potentially modifying its mechanical properties, corrosion resistance, and fatigue behavior. The HAZ, in particular, can exhibit markedly different characteristics from the base metal and weld metal.
3. Material Property Alterations:
Welding can significantly alter the properties of the base material. These changes may include modifications to strength, ductility, toughness, and hardness. In some alloys, precipitation hardening or grain growth can occur, while in others, softening due to over-aging might be observed. Understanding and mitigating these metallurgical changes is crucial for maintaining the desired material properties in the welded structure.
4. Stress Concentration and Failure Modes:
Welding stress, particularly residual stress, acts as a significant contributor to various failure mechanisms:
5. Dimensional Inaccuracies:
Welding distortion manifests as changes in the shape and dimensions of the welded structure. This can lead to:
These distortions can significantly impact the manufacturing quality, functional performance, and service life of the welded structure. Mitigation strategies such as proper joint design, controlled heat input, sequencing of welds, and post-weld treatments are often necessary to minimize these adverse effects.
1. Distortion
Distortion in welding refers to the unintended alteration in the shape and dimensions of a workpiece, resulting from the non-uniform heating and cooling cycles inherent in the welding process. This phenomenon occurs due to the localized thermal expansion and contraction of the material, as well as phase transformations in the heat-affected zone (HAZ).
2. Stress
Stress is defined as the internal force per unit area acting within a material in response to external loads or other factors such as thermal gradients. In welding, stress manifests as:
3. Welding stress and welding distortion
Welding stress refers to the complex system of internal stresses that develop within a weldment during and after the welding process. These stresses arise from:
Welding distortion is the measurable change in the geometry and dimensions of a weldment caused by the cumulative effects of welding stresses. Common types of welding distortion include:
Understanding the interrelationship between welding stress and distortion is crucial for implementing effective mitigation strategies in welding design and fabrication processes.
1. Uneven heating of weldments
(1) Stress and distortion caused by central heating of long strip (similar to surfacing)
Stress and distortion of steel strip center during heating and cooling
(2) Stress and distortion caused by heating on one side of the long strip (equivalent to plate edge surfacing)
Stress and distortion during heating and cooling on one side of steel plate edge
2. Shrinkage of welded metal
3. Change of metal structure
4. Rigidity and restraint of weldment
Welding distortion can be divided into five basic forms: shrinkage distortion, angular distortion, bending distortion, wave distortion and deformation distortion.
Basic forms of welding distortion
1). Shrinkage distortion
The phenomenon that the size of weldment is shorter than that before welding is called shrinkage distortion.
Longitudinal and transverse shrinkage distortion
(1) Longitudinal shrinkage distortion
(2) Transverse shrinkage distortion
2). Angular distortion
The root cause of angular distortion is the uneven distribution of transverse shrinkage along the plate thickness.
Angular distortion of several joints
Angular distortion of T-joint
3). Bending distortion
Bending distortion is caused by the non-coincidence or asymmetry between the centerline of the weld and the neutral axis of the structural section, as well as the uneven distribution of the shrinkage of the weld along the width of the weldment.
(1) Bending distortion caused by longitudinal shrinkage
Bending distortion caused by longitudinal shrinkage of weld
(2) Bending distortion caused by transverse shrinkage
Bending distortion caused by transverse shrinkage of the weld
4). Wave distortion
Wave distortion often occurs in the welding process of thin plates with a thickness of less than 6mm, which is also called instability distortion.
Wave distortion caused by weld fillet distortion
5). Distortion
The main cause of distortion is the uneven distribution of weld fillet distortion along the weld length.
Distortion of I-beam
1). Design measures
(1) Select a reasonable weld shape and size
1) Select the smallest weld size.
Cross joint with the same bearing capacity
2) Select a reasonable groove form.
Groove of T-joint
(2) Reduce the number of welds
Profiles and stamping parts are the preferred options when possible. For structures with many and dense welds, cast weld joint structures can be used to reduce the number of welds. Additionally, increasing the thickness of the wall plate to reduce the number of ribs, or using profiled structures instead of rib structures, can help prevent distortion of thin plate structures.
(3) Reasonable arrangement of weld position
Beam, column and other welded components often have bending distortion due to the eccentric configuration of the weld.
Weld arrangement of the box structure
Reasonably arrange the weld position to prevent distortion
2). Process measures
(1) Allowance method
(2) Inverse distortion method
Inverse distortion method for flat plate butt welding
(3) Rigid fixation method
1) Fix the weldment on the rigid platform.
Rigid fixation during thin plate splicing
2) The weldment is combined into a more rigid or symmetrical structure.
Rigid fixation and anti-distortion of T-beam
3) The welding fixture is used to increase the rigidity and restraint of the structure.
Rigid fixation during butt splicing
4) Use temporary supports to increase the restraint of the structure.
Temporary support during shield welding
(4) Select a reasonable assembly and welding sequence.
The assembly welding sequence has a great influence on the distortion of the welded structure.
(1) If conditions permit, large and complex welded structures should be divided into several parts with simple structures, welded separately, and then assembled as a whole.
(2) The weld when welding should be close to the neutral axis of the structural section as much as possible.
Assembly and welding of the main beam
3) For the structure with the asymmetric arrangement of welds, the side with few welds shall be welded first during assembly welding.
Welding sequence of upper die of press
4) The structure with a symmetrical arrangement of welds shall be welded symmetrically by even welders.
Welding sequence of cylinder butt weld
5) When welding long welds (more than 1m), the direction and sequence shown in the figure below can be used to reduce the shrinkage distortion after welding.
(5) Reasonably select welding methods and welding process parameters
Welding of asymmetric section structure
(6) Heat balance method
Use the heat balance method to prevent welding distortion
(7) Heat dissipation method
1). Manual correction
2). Mechanical correction method
Correction of bending distortion of the beam by the mechanical correction method
3). Flame heating correction method
The ways of flame heating include point heating, linear heating and triangular heating.
(1) Spot heating
(2) Linear heating
(3) Triangular heating
Flame correction of bending distortion of I-beam
The correction of welding distortion by flame heating depends on the following three factors:
(1) Heating mode
(2) Heating position
(3) Heating temperature and area of the heating zone
1). According to the causes of stress
(1) Thermal stress
Thermal stress arises from non-uniform temperature distribution during the welding process. As the weld metal and surrounding base material heat up and cool down at different rates, localized expansion and contraction occur, leading to stress development.
(2) Transformation stress
Transformation stress, also known as phase transformation stress, results from volume changes associated with microstructural alterations in the material during heating and cooling cycles. This is particularly significant in steels undergoing martensitic or bainitic transformations.
(3) Plastic strain stress
Plastic strain stress develops when the material experiences localized yielding due to thermal gradients and constraints during welding. This non-uniform plastic deformation contributes to the residual stress state after cooling.
2). According to the time of stress existence
(1) Welding transient stress
Welding transient stress, also referred to as instantaneous stress, occurs during the welding process itself. It is a dynamic stress state that evolves rapidly as the heat source moves along the weld path, causing continuous changes in temperature distribution and material properties.
(2) Welding residual stress
Welding residual stress is the static stress that remains in the welded structure after it has completely cooled to ambient temperature and all external loads have been removed. This stress can significantly impact the mechanical behavior and service life of welded components.
1). Distribution of longitudinal residual stress σx
Distribution of butt joint on weld 0x cross section
2). Distribution of transverse residual stress σy
(1) The transverse stress caused by longitudinal shrinkage of welding and its adjacent plastic distortion zone is σ’y
(2) Mechanical stress caused by transverse shrinkage year σ” y
Distribution of σ” Y during welding in different directions
1). Impact on structural strength
2). Influence on the dimensional accuracy of weldment processing
Internal stress release and distortion caused by machining
3). Influence on the stability of compression members
1). Design measures
1) Minimize the number and size of welds on the structure.
2) Avoid excessive concentration of welds, and keep sufficient distance between welds.
Welding of vessel nozzle
3) The joint form with less rigidity shall be adopted.
Measures to reduce the rigidity of joints
2). Process measures
1) Adopt reasonable assembly welding sequence and direction.
① When welding the weld on a plane, it shall be ensured that the longitudinal and transverse shrinkage of the weld can be relatively free.
Reasonable assembly and welding sequence of splicing welds
② The weld with the largest shrinkage shall be welded first.
Welding sequence of duplex beam structure with cover plate
③ The weld with the largest stress during operation shall be welded first.
Welding sequence of butt I-beam
④ When the plane cross weld is welded, it is easy to produce large welding stress at the intersection of the weld.
Welding sequence of plane cross welds
⑤ The structure where butt welds and fillet welds intersect.
2) Preheating method.
3) Cold welding.
4) Reduce the restraint of welds.
Reduce local stiffness and internal stress
Schematic diagram of heating “stress relief zone” method
(1) Integral heat treatment
(2) Local heat treatment
2). Mechanical stretching method
3). Temperature difference stretching method
Schematic diagram of eliminating residual stress by “temperature difference tensile method”
4). Hammer weld
5). Vibration method
1). Mechanical Methods
(1) Sectioning Method
The sectioning method involves carefully cutting the welded component into smaller pieces, allowing for the release and measurement of residual stresses. This destructive technique provides a comprehensive stress profile across the weld and heat-affected zone (HAZ).
(2) Hole-Drilling Method
This semi-destructive method involves drilling a small hole in the welded area and measuring the resulting strain relaxation. It’s particularly effective for near-surface residual stress measurement and can provide stress distribution data at various depths.
2). Non-Destructive Physical Methods
(1) Magnetic Methods
Techniques such as Barkhausen Noise Analysis (BNA) and Magnetostriction exploit the relationship between a material’s magnetic properties and its stress state. These methods are particularly suitable for ferromagnetic materials and can provide rapid, on-site measurements.
(2) X-ray Diffraction (XRD)
XRD utilizes the principle of Bragg’s Law to measure lattice deformations caused by residual stresses. This highly accurate method is non-destructive and can measure surface stresses with excellent spatial resolution, making it ideal for complex geometries and multi-pass welds.
(3) Ultrasonic Methods
These techniques leverage the acoustoelastic effect, where the velocity of ultrasonic waves changes with the stress state of the material. Time-of-flight diffraction (TOFD) and critically refracted longitudinal (LCR) waves are commonly used for through-thickness residual stress measurement in thick welded components.
(4) Neutron Diffraction
While not mentioned in the original list, neutron diffraction is a powerful non-destructive method for measuring residual stresses deep within thick welded components. It offers excellent penetration depth and is particularly useful for complex geometries and multi-phase materials.
Uniform heating of the entire part before welding can significantly reduce thermal differences that cause distortion. This can be achieved using ovens or other controlled heating mechanisms, and ensuring even cooling by hanging the component vertically with good air flow is also crucial to prevent differential contraction.
Preheating the metal before welding helps reduce temperature differences between the weld area and the rest of the workpiece. This minimizes abrupt expansion and contraction, thereby reducing the potential for distortion.
Strong clamps can hold parts in place during welding, preventing movement and maintaining alignment. Applying clamps uniformly is important to avoid introducing uneven stresses. Specialized clamps, such as heat-sink clamps, can also help manage heat distribution.
Custom fixtures and jigs can hold parts in precise positions during welding, ensuring they do not move and reducing the risk of distortion. These tools are particularly useful for repetitive welding tasks in production environments.
Stitch welding uses a series of short welds instead of a continuous weld. This technique reduces the amount of heat input in a localized area, helping to prevent excessive thermal expansion and contraction, especially in thin materials prone to warping.
Backstep welding changes the direction of weld deposition, starting from the end of the joint and moving backward to balance thermal stresses and reduce distortion. This technique is effective in managing shrinkage forces.
Peening the weld bead with a hammer stretches the metal and relieves stresses, especially useful in reducing distortion in butt and corner welds. Care must be taken to avoid over-peening, which can cause surface defects.
Heat-sink clamps, such as the Dimide 1/4 Series Clamp, use aluminum jaws with cooling fins to pull heat away, reducing distortion and allowing the weld to cool more evenly.
Machines from companies like SEC Industrial achieve precise, distortion-free welds by closely controlling the welding process. These machines are particularly useful for complex welds in pipeline construction and other industrial applications.
Ensuring tight fit-up of metal parts before welding minimizes gaps and reduces the weld metal needed. Proper part fit-up is essential for maintaining the structural integrity and alignment of the welded assembly.
Cleaning metal surfaces before welding improves weld quality and reduces the risk of distortion and defects. Contaminants can affect the weld pool and lead to uneven heating and cooling.
Controlled heating and cooling of the weldment reduce residual stresses by achieving a more uniform stress distribution. This process involves heating the weldment to an elevated temperature and then cooling it in a controlled manner.
Copper chill bars near the weld area absorb excess heat, preventing overheating and reducing distortion, thanks to copper’s high thermal conductivity.
By implementing these practical tips and utilizing the right tools, welders can effectively manage and minimize distortion, ensuring high-quality and structurally sound welded components.
Below are answers to some frequently asked questions:
The primary causes of weld distortion include uneven heating and cooling, residual stress, and thermal expansion and contraction. Uneven heating and cooling during welding cause differential thermal expansion and contraction, leading to distortion. Residual stress arises from the compressive and tensile stresses created in the parent metal and weld metal during heating and cooling. The inherent thermal expansion and contraction of the weld pool as it heats and cools also generate stresses that contribute to distortion. Additionally, factors such as the welding technique and process, material properties, joint preparation, weld size, fixturing and clamping, and heat input can significantly impact the extent of weld distortion. Understanding these causes is crucial for implementing effective strategies to minimize distortion and ensure the structural integrity of welded components.
Thermal expansion and contraction significantly affect weld distortion due to the changes in temperature during the welding process. When metal is heated, it expands. This expansion is resisted by the surrounding cooler metal, creating internal stresses. As the weld cools and solidifies, the metal contracts, causing shrinkage in the weld area and the heat-affected zone (HAZ). This contraction is also resisted by the cooler parent metal, leading to tensile and compressive stresses that can exceed the metal’s yield strength, resulting in permanent deformation and distortion. This distortion can manifest in various forms, such as longitudinal, transverse, or angular distortion, depending on the welding conditions and the material properties.
The different types of weld distortion include longitudinal distortion, transverse distortion, angular distortion, buckling, bowing, warping, rotational distortion, and twisting distortion. Longitudinal distortion occurs along the length of the weld, causing the workpiece to shorten. Transverse distortion happens perpendicular to the welding seam, pulling the edges of the joint together. Angular distortion alters the angles between welded parts due to non-uniform transverse shrinkage. Buckling, bowing, and warping are complex distortions that involve multiple types happening simultaneously, leading to the metal curving or dishing inward. Rotational distortion involves a twisting movement around the welding line, while twisting distortion results from uneven heating and cooling, causing the workpiece to twist. These distortions are influenced by factors such as restraint, material properties, weld design, heat input, and the welding procedure.
To design weld joints and minimize distortion, several strategies can be employed. Ensure the weld joint is correctly sized to avoid overwelding, which increases shrinkage forces. Place welds near the neutral axis and balance them on both sides to reduce leverage effects. Plan a careful welding sequence, alternating sides to counteract shrinkage forces. Use intermittent welding and minimize the number of weld passes. Clamping and fixtures help maintain alignment, while presetting parts accounts for shrinkage. Thermal stress relief techniques can reduce residual stresses. Optimize welding parameters to reduce heat input, and consider design choices that minimize the amount of weld metal needed. These approaches collectively help in reducing weld distortion.
To reduce distortion in welding, several techniques can be employed:
By incorporating these techniques, welders can significantly reduce distortion, resulting in more accurate and stable weldments.
The choice of shielding gas significantly affects weld distortion by influencing the thermal properties of the weld metal, heat distribution, arc stability, and overall weld geometry. The composition of the shielding gas, such as the proportions of argon, helium, carbon dioxide, and oxygen, can alter the cooling rates and thermal conductivity, which in turn impacts the degree of transverse and angular shrinkage. For example, a higher percentage of argon can slow down cooling, increasing shrinkage, whereas helium can speed up cooling and reduce shrinkage. Additionally, optimizing the gas flow rate helps maintain consistent heat input, thereby minimizing distortion. Therefore, selecting the appropriate shielding gas composition and maintaining optimal flow rates are crucial for controlling weld distortion and achieving high-quality welds.