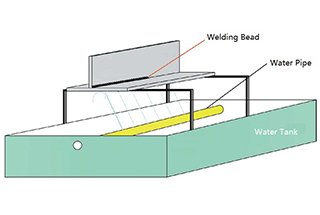
Ever wondered how tiny particles can make or break your electronic devices? In this article, we explore the fascinating world of welding flux, the unsung hero in welding and soldering. Learn how this crucial material ensures strong, clean welds and what it means for the quality of your electronics. Get ready to dive into the science behind seamless connections!
Welding flux is a crucial auxiliary material in the welding process, primarily composed of complex chemical compounds designed to enhance weld quality and protect the weld pool. When heated during welding, this granular substance melts to form protective slag and shielding gases, playing a vital role in metallurgical treatment and weld integrity.
The composition of welding flux varies depending on the specific welding application, but typically includes a mixture of minerals, silicates, carbonates, fluorides, and deoxidizers. In some applications, such as electronics assembly, flux may contain rosin or other organic compounds. This carefully formulated blend serves multiple critical functions:
The quality and composition of the flux directly impact weld integrity, corrosion resistance, and overall product performance. In electronic assembly, where precision and cleanliness are paramount, specialized low-residue or no-clean fluxes are often employed to minimize post-weld cleaning requirements while still ensuring high-quality solder joints.
Proper selection and application of welding flux, tailored to the specific base materials, welding process, and end-use requirements, is essential for achieving optimal weld quality and meeting stringent industry standards.
The welding flux is composed of a mixture of minerals, including marble, quartz, fluorite, and others, as well as chemicals such as titanium dioxide and cellulose.
The primary application of welding flux is in submerged arc welding and electroslag welding.
Related reading: Types of welding
In the past few decades, in the soldering process of electronic product production, rosin resin flux composed mainly of rosin, resin, halide-containing activators, additives, and organic solvents are often used.
Although this type of flux has good solderability and low cost, it has high post-weld residues. These residues contain halide ions, which gradually cause problems such as decreased electrical insulation performance and short-circuits.
To solve this problem, it is necessary to clean the rosin resin flux residues on the electronic printed board, which not only increases production costs, but also the solvent used to clean the rosin resin flux residues is mainly fluorochloro compounds. This compound is a substance that depletes the atmospheric ozone layer and is currently banned and phased out.
However, for various reasons, many companies still use the process of soldering with rosin resin flux and then cleaning with a fluorochlorine cleaner, which has low efficiency and high cost, and causes serious environmental pollution.
The no-clean flux, which is used more in the market and is of a higher grade, is composed of: organic solvents, natural resins and their derivatives, synthetic resin surfactants, organic acid activators, anti-corrosive agents, co-solvents, and film-forming agents.
Simply put, it is a homogeneous transparent mixed solution formed by dissolving various solid components in various liquids, where each component has different proportions and functions.
Organic solvents:
A mixture of one or several types of ketones, alcohols, esters, commonly used ones include ethanol, propanol, butanol; acetone, toluene isobutyl ketone; ethyl acetate, butyl acetate, etc.
As a liquid component, its main function is to dissolve the solid components in the flux to form a homogeneous solution, making it easier for the components to be soldered to evenly coat an appropriate amount of flux components, while it can also clean light dirt and oil on the metal surface.
Constituent Components | Primary Function | |
Volatile Components | Solvent | Regulation of viscosity and dispersion of solid components |
Solid Composition | Resin | Primary ingredients, catalytic soldering functions |
Dispersant | Prevention of separation, fluidity characteristics | |
Activator | Deoxidation |
Natural Resin and its Derivatives or Synthetic Resin Surfactants:
Halogen-containing surfactants have high activity and soldering ability, but because halogen ions are difficult to clean, ion residues are high, and halogen elements (mainly chlorides) have strong corrosive properties, they are not suitable for use as raw materials for no-clean flux.
Non-halogen-containing surfactants have slightly weaker activity, but fewer ion residues. Surfactants are mainly non-ionic surfactants of the fatty acid family or aromatic family. Their main function is to reduce the surface tension generated when the solder contacts the metal of the lead, enhance the surface wetting force, enhance the penetration of organic acid activators, and also play a role as a foaming agent.
Organic Acid Activator:
Composed of one or several kinds of dicarboxylic acids or aromatic acids, such as succinic acid, glutaric acid, itaconic acid, salicylic acid, fumaric acid, heptanoic acid, malic acid, succinic acid, etc., its main function is to remove oxides on the lead foot and the surface of the molten solder, and it is one of the key components of the flux.
Anti-corrosive Agent:
Reduces the residues of solid components such as resins and activators after high-temperature decomposition.
Co-solvent:
Prevents the tendency of solid components such as activators to desorb from the solution, avoiding the poor uniform distribution of activators.
Film-forming Agent:
During the soldering process of the lead, the applied flux precipitates and crystallizes to form a uniform film. The residues after high-temperature decomposition can be quickly solidified, hardened, and reduced in stickiness due to the presence of the film-forming agent.
The working principle of flux is fundamental to achieving high-quality welds in various metalworking processes. Flux plays a crucial role in removing surface oxides, enhancing wettability, and promoting strong metallurgical bonds between the base metal and filler material.
During the welding process, flux actively removes the oxide layer from the surface of the welding material through chemical reactions. This cleaning action is primarily performed by activators within the flux composition. These activators, typically halide compounds or organic acids, rapidly break down and dissolve metal oxides, creating a clean surface for bonding.
Simultaneously, flux reduces the surface tension between the molten solder and the base metal. This reduction in surface tension is achieved through the action of surfactants present in the flux formulation. By lowering surface tension, flux significantly enhances the flow characteristics and wetting properties of the liquid solder, allowing it to spread evenly and penetrate even the smallest gaps in the joint.
The multi-faceted action of flux can be broken down into several key functions:
In wave soldering applications, such as those used in electronics manufacturing, the flux’s wetting action becomes particularly evident. As the soldered component exits the molten solder wave, the flux-induced wetting causes excess solder to flow smoothly down the leads or pins. This action helps prevent common defects like solder bridging between adjacent connections or the formation of sharp solder spikes.
The effectiveness of flux is highly dependent on its composition, which is tailored to specific welding processes, base metals, and operating temperatures. Modern flux formulations often incorporate advanced additives to enhance performance, reduce environmental impact, and comply with increasingly stringent manufacturing regulations.
Functions of the flux:
(1) Remove oxides and contaminants from the welding surface, reducing the melting point and surface tension of the filler metal. This facilitates rapid achievement of the optimal brazing temperature, enhancing process efficiency.
(2) Create a protective atmosphere around the molten weld pool, shielding it from detrimental atmospheric gases such as oxygen and nitrogen, which could otherwise lead to porosity or embrittlement.
(3) Optimize the viscosity and flow characteristics of the liquid filler metal, ensuring proper capillary action and complete filling of the joint gap, crucial for producing high-quality, leak-free brazed connections.
(4) Chemically react with and dissolve metal oxides on the base material and filler metal surfaces, promoting effective wetting and facilitating the formation of strong metallurgical bonds at the joint interface.
(5) Form a protective slag layer over the solidifying weld metal, preventing re-oxidation during cooling and contributing to the overall integrity of the joint.
(6) Enhance the surface activity of both the filler metal and the base materials by reducing interfacial tensions, thereby improving wettability and spreading of the molten filler.
(7) Modify the rheological properties of the molten filler metal, often through the formation of a flux-metal eutectic mixture, further enhancing flowability and wetting characteristics.
(8) Act as a thermal transfer medium, improving heat distribution from the heat source (e.g., torch, induction coil, or furnace) to the joint area, ensuring more uniform heating and reducing the risk of localized overheating.
(9) Contribute to the aesthetic quality of the finished joint by promoting smooth fillet formation and reducing surface irregularities, which can be particularly important in visible or cosmetic applications.
(10) In some advanced fluxes, incorporate alloying elements that can diffuse into the joint, potentially enhancing mechanical properties or corrosion resistance of the brazed connection.
Functions of the flux in submerged arc welding:
(1) Mechanical and chemical protection: During arc welding, the flux melts to form a protective slag layer over the weld pool. This layer shields the molten metal from atmospheric gases, preventing oxidation and nitrogen absorption, which could lead to porosity and embrittlement in the weld. The flux also provides a stable gaseous environment for arc maintenance.
(2) Metallurgical refinement: The flux transfers alloying elements to the weld pool, adjusting its chemical composition. It can also remove impurities through slag-metal reactions, improving the mechanical properties and quality of the weld.
(3) Weld bead shaping and stabilization: The flux promotes the formation of a smooth, uniform weld bead with good penetration and minimal spatter. The viscosity and surface tension of the molten flux help control the weld pool dynamics and solidification behavior.
The melting point of the flux should typically be 10-30°C lower than that of the base metal and filler wire. In special applications, flux with a higher melting point may be used. If the flux melting point is significantly lower than the base metal, it may prematurely melt and lose its effectiveness due to vaporization or premature reactions.
Flux selection is largely dependent on the oxide film characteristics of the base metal:
Fluoride-based fluxes are also common, reacting uniformly and leaving minimal residue. For instance, boron trifluoride (BF3) mixed with nitrogen is used for high-temperature brazing of stainless steel.
For lower temperature brazing (below 450°C), soft fluxes are employed:
(1) Melting point characteristics:
The flux must have a melting point lower than that of the solder alloy. This ensures that the flux becomes active and performs its functions before the solder melts, preparing the surfaces for optimal bonding.
(2) Physical properties in relation to solder:
The flux should possess lower surface tension, viscosity, and density compared to the solder. These properties allow the flux to spread easily across the joint area and be displaced by the molten solder, ensuring complete coverage and minimizing the risk of flux entrapment.
(3) Chemical compatibility and functionality:
An ideal flux should not corrode the base materials being joined. It must enhance the flowability of the solder while effectively removing oxide films from metal surfaces at the soldering temperature. This dual action promotes better wetting and stronger metallurgical bonds.
(4) Post-process considerations:
Flux residues should be easily removable after the soldering process. This characteristic is crucial for ensuring the cleanliness of the final product, preventing potential long-term reliability issues, and facilitating subsequent manufacturing steps or surface treatments.
(5) Health and environmental safety:
The flux should not produce toxic gases or strong odors during the soldering process. This requirement is essential for protecting workers’ health and preventing environmental pollution. It aligns with modern manufacturing standards that prioritize workplace safety and environmental responsibility.
There are several ways to classify welding flux, including its usage, method of manufacture, chemical composition, metallurgical properties during welding, and the pH and particle size of the flux.
Regardless of the classification method used, it only highlights certain aspects of the welding flux and does not fully encompass all its characteristics.
Common classification methods include:
A neutral welding flux is a specialized consumable that maintains the chemical integrity of both the deposited metal and the welding wire during the welding process. This flux type is engineered to minimize chemical interactions, ensuring that the weld metal composition closely matches that of the base metal and filler material.
Neutral fluxes are particularly advantageous in multi-pass welding applications, especially when working with thick-section materials exceeding 25 mm. Their non-reactive nature makes them ideal for maintaining consistent mechanical properties throughout the weld, which is crucial for structural integrity in heavy fabrication industries such as shipbuilding, pressure vessel manufacturing, and large-scale infrastructure projects.
Key characteristics of neutral welding fluxes include:
a. Minimal oxide content: Unlike active fluxes, neutral fluxes contain negligible amounts of oxides such as SiO2, MnO, and FeO. This composition helps prevent undesired alloying reactions during welding.
b. Non-oxidizing properties: The flux formulation is designed to create an inert atmosphere around the weld pool, effectively shielding it from atmospheric oxygen and preventing oxidation of the weld metal.
c. Sensitivity to base metal condition: When welding on heavily oxidized base metals, neutral fluxes may not provide sufficient cleaning action. This can lead to potential weld defects such as porosity and cracking in the weld bead. Therefore, proper surface preparation is critical when using neutral fluxes.
d. Consistent arc stability: Neutral fluxes promote stable arc characteristics, facilitating smooth metal transfer and uniform weld bead formation.
e. Excellent slag detachability: The slag formed by neutral fluxes typically separates easily from the weld surface, reducing post-weld cleaning requirements and improving productivity.
f. Controlled heat input: These fluxes often help in maintaining optimal heat input levels, which is crucial for controlling grain structure and minimizing heat-affected zone (HAZ) in multi-pass welds.
When selecting a neutral welding flux, it’s essential to consider factors such as the base metal composition, welding parameters, and specific application requirements to ensure optimal weld quality and performance.
An active welding flux is a specialized compound containing small quantities of deoxidizing agents, primarily manganese (Mn) and silicon (Si). This type of flux plays a crucial role in enhancing weld quality by improving resistance to porosity and cracking, two common defects in welding processes.
The key characteristics of an active welding flux include:
a. Chemical Composition Influence: The presence of deoxidizers like Mn and Si can significantly impact the chemical composition of the deposited weld metal. This effect is particularly sensitive to fluctuations in arc voltage. As the arc voltage increases, more Mn and Si are transferred to the weld pool, resulting in:
b. Enhanced Porosity Prevention: Active welding fluxes exhibit superior capability in preventing porosity. This is achieved through two primary mechanisms:
c. Improved Weld Bead Appearance: The active components in the flux contribute to better wetting and spreading of the molten metal, resulting in smoother weld bead profiles and improved fusion with the base metal.
d. Slag Detachability: Active fluxes typically produce a slag that is easily removable after welding, facilitating post-weld cleaning and inspection processes.
e. Versatility: Active welding fluxes are suitable for a wide range of base metals and welding positions, making them a versatile choice for many industrial applications.
An alloy welding flux is a specialized welding consumable that contains additional alloying elements, primarily serving as transition metals. These fluxes are predominantly manufactured through a sintering process, which involves compacting and heating the powder mixture below its melting point to create a solid, cohesive mass.
The primary applications of alloy welding fluxes include:
Key features and benefits of alloy welding fluxes include:
When selecting an alloy welding flux, considerations such as base metal composition, desired weld properties, and specific application requirements must be carefully evaluated to ensure optimal performance and weld quality.
Melting welding flux is produced through a precise metallurgical process involving the careful combination of various mineral raw materials. These materials are proportioned according to specific formulations to achieve desired welding characteristics. The mixture is then subjected to high-temperature processing, typically exceeding 1300°C, which causes the components to melt and homogenize thoroughly.
The molten flux undergoes rapid quenching in water, resulting in the formation of glassy granules. This quenching process not only shapes the flux but also imparts specific properties crucial for its welding performance. The granules are then dried to remove moisture, crushed to achieve the desired particle size distribution, and sieved to ensure uniformity. Finally, the processed flux is packaged for distribution and use in welding applications.
In China, a widely recognized classification system for melting welding flux is the “HJ” series. This alphanumeric designation provides valuable information about the flux composition:
This standardized system allows welders and engineers to quickly identify the key properties of the flux, facilitating proper selection for specific welding applications and base materials.
After precise proportioning of raw materials, the ingredients undergo a two-stage mixing process. Initially, dry mixing ensures uniform distribution of components. Subsequently, a binder, typically sodium silicate (water glass), is introduced for wet mixing to achieve optimal cohesion and plasticity.
The homogenized mixture then enters a granulation phase, where it’s formed into particles of specific size and shape to enhance flux performance and handling characteristics. This granulated material is conveyed to a drying oven for controlled curing and moisture removal, crucial for flux stability.
The final stage involves sintering at approximately 500°C (932°F). This high-temperature process facilitates partial fusion of the granules, improving their strength, density, and chemical reactivity. The sintering temperature is carefully regulated to maintain the desired flux properties without compromising its melting behavior during welding.
In China, sintered welding fluxes are commonly designated by the “SJ” prefix, followed by a three-digit code. The first digit after “SJ” indicates the primary slag system, which determines the flux’s fundamental chemical and physical properties. The subsequent two digits differentiate between various brands or formulations within the same slag system, often reflecting specific performance characteristics or intended applications.
For instance, SJ101 and SJ102 would belong to the same slag system (indicated by “1”) but may have slight variations in composition or properties to suit different welding conditions or materials.
Flux types can be broadly categorized into organic, inorganic, and resin-based varieties, each with distinct properties and applications in metal joining processes.
Resin-based flux, primarily derived from tree secretions, is a natural product known for its low corrosivity. Rosin, the most prominent example of this category, has lent its name to the term “rosin flux.” Its mild nature makes it particularly suitable for electronics and other sensitive applications where minimal residue and post-soldering cleaning are crucial.
Flux classification can also be based on its compatibility with different solder types, resulting in the distinction between soft and hard fluxes. This categorization aligns with the properties of the solder being used, ensuring optimal performance in various joining operations.
In the assembly and maintenance of electronic products, several soft flux types are commonly employed:
The selection of an appropriate flux type depends on various factors, including the base metals being joined, the specific soldering application, environmental conditions, and post-soldering cleaning requirements. For instance, no-clean fluxes are preferred in situations where post-soldering cleaning is impractical, while more active fluxes might be necessary for joining difficult-to-solder metals or heavily oxidized surfaces.
It’s crucial to consider factors such as flux activation temperature, cleaning methods, potential for corrosion, and compatibility with the soldering process when choosing the most suitable flux for a given application. This ensures optimal joint quality, reliability, and long-term performance of the soldered assembly.
Control of flux drying and heat preservation
Prior to use, flux must be baked according to manufacturer specifications. These drying parameters are derived from rigorous testing and process control, resulting in quality-assured, validated data. While these form enterprise standards, requirements may vary between organizations.
For reference, JB4709-2000 “Welding Procedure for Steel Pressure Vessels” provides recommended flux drying temperatures and retention times. Generally, when drying flux, the pile height should not exceed 5cm. Welding material storage often involves drying larger quantities, favoring thicker layers over thin ones. Strict adherence to these guidelines is crucial to ensure thorough flux drying quality.
To prevent inadequate drying due to excessive stacking, extend drying times proportionally to flux layer thickness. Implement a systematic approach to flux drying, considering factors such as ambient humidity, flux composition, and intended application.
Control of flux on-site management and recycling disposal
Maintain a clean welding area to prevent debris contamination of the flux. Flux for padding should be dispensed as per regulations, ideally kept at approximately 50°C. Implement timely flux recycling to minimize contamination risks.
For reused flux, employ an 8-40 mesh sieve system to remove impurities and fine powder. Blend this with new flux at a 1:3 ratio (used:new). Before reuse, dry the mixture at 250-350°C for 2 hours, then store in a 100-150°C holding oven until needed. Strictly prohibit outdoor storage.
In challenging field conditions or high humidity environments, implement rigorous site management practices. Regularly test flux for moisture resistance and mechanical inclusions. Control moisture absorption rates and mechanical contaminants. Avoid haphazard stacking and unintended flux mixing.
Flux particle size and distribution requirements
Flux particle size significantly impacts welding performance. Optimal sizing ensures proper flux permeability, minimizes continuous arc emissions, and prevents air entrapment in the weld pool, which can lead to porosity. Fluxes typically fall into two categories:
Generally, fine powder below the specified size should not exceed 5%, while coarse particles above the specified size should be less than 2%. Regular particle size distribution analysis is essential to determine appropriate welding current parameters and ensure consistent weld quality.
Control of flux layer thickness and heap scatter height
Precise control of flux layer thickness is critical, with the optimal range typically between 25-40mm. Insufficient or excessive flux layers can lead to surface defects such as pits, spots, and pores, resulting in irregular weld bead geometry.
When using sintered flux, due to its lower density, increase the pile height by 20-50% compared to fused flux. Adjust flux layer thickness proportionally to wire diameter and welding current: larger wire diameters and higher currents require thicker flux layers.
Non-standard welding procedures or improper handling of fine powder flux can result in intermittent surface imperfections. While these may pass non-destructive testing, they can compromise appearance quality and locally reduce shell thickness. Implement strict process controls and proper flux management to mitigate these issues and ensure consistent, high-quality welds.
Selecting the appropriate flux is crucial for achieving high-quality welding results. While users cannot typically perform comprehensive chemical analyses, there are several practical methods to evaluate and choose the right flux:
What is a flux in welding?
Flux in welding is a chemical agent used to promote fusion, protect the weld pool, and enhance the quality of the final weld. It serves multiple critical functions: preventing oxidation, removing impurities, stabilizing the arc, and improving weld penetration. Flux can be applied as a coating on electrodes (stick welding), contained within tubular wire (flux-cored arc welding), or as a separate granular material (submerged arc welding). By creating a protective atmosphere and slag layer, flux ensures a cleaner, stronger, and more uniform weld joint.
Do you need flux for stick welding?
Yes, flux is integral to stick welding (Shielded Metal Arc Welding – SMAW). The welding electrode in SMAW is coated with a flux material. As the electrode melts during welding, the flux coating vaporizes, creating a protective gas shield around the weld pool. This shield displaces atmospheric gases, preventing contamination from oxygen and nitrogen. Additionally, the flux forms a slag layer that further protects the cooling weld and helps shape the bead. The flux also contributes alloying elements to the weld, influencing its mechanical properties and chemical composition.
Is flux welding as strong as MIG?
The strength of a weld depends on multiple factors, including base material, filler metal selection, welding parameters, and welder skill, rather than solely on the welding process. Both flux-cored arc welding (FCAW) and gas metal arc welding (GMAW/MIG) can produce high-quality, strong welds when executed properly. FCAW often excels in outdoor applications and on thicker materials due to its superior penetration and tolerance for contaminants. MIG welding typically offers cleaner welds with less post-weld cleanup and is preferred for thin materials and aesthetically critical applications. The choice between FCAW and MIG should be based on specific project requirements, material thickness, and welding environment.
What is flux welding best for?
Flux welding, particularly flux-cored arc welding (FCAW), excels in several applications:
FCAW combines the simplicity of stick welding with the productivity of wire-fed processes, making it a versatile choice for many industrial and construction applications. However, proper ventilation is crucial due to the fumes generated by the flux.