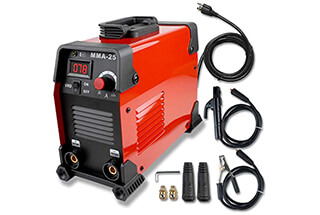
Welding low-temperature steel requires a keen understanding of material properties, as extreme conditions can lead to brittle failures. The article discusses the technical requirements for low-temperature steel, emphasizing the importance of low-temperature toughness and the role of various elements and heat treatments in achieving it. Additionally, it covers welding methods, material selection, and specific practices for welding different types of low-temperature steel, such as A333-GR6. Readers will gain insights into ensuring weld quality and preventing defects in these critical applications.
1) The critical technical requirements for low-temperature steel encompass sufficient strength, ample toughness under cryogenic conditions, excellent weldability, good machinability, and superior corrosion resistance.
Among these, low-temperature toughness—the ability to resist brittle fracture at subzero temperatures—is paramount. Consequently, most national standards specify minimum impact toughness values at the lowest service temperatures, typically measured using Charpy V-notch tests.
2) In the composition of low-temperature steel, elements such as carbon, silicon, phosphorus, sulfur, and nitrogen are generally detrimental to low-temperature toughness, with phosphorus being the most deleterious.
To mitigate this, phosphorus removal is prioritized during early stages of steelmaking, often through secondary metallurgy processes like vacuum degassing. Conversely, elements like manganese and nickel enhance low-temperature toughness. Each 1% increase in nickel content can lower the ductile-to-brittle transition temperature (DBTT) by approximately 20°C, primarily by stabilizing the austenite phase and refining grain structure.
3) The heat treatment process plays a crucial role in determining the microstructure and grain size of low-temperature steel, significantly influencing its cryogenic toughness. Quenching and tempering treatments, when optimized, can markedly improve low-temperature toughness by producing a fine-grained martensitic or bainitic structure with controlled precipitation of carbides.
4) Based on primary forming methods, low-temperature steel can be classified into cast steel and wrought (rolled) steel.
Depending on composition and microstructural characteristics, low-temperature steels are categorized as: low-alloy steels (e.g., ASTM A353, A553), 3.5% Ni steels, 5% Ni steels, 6% Ni steels, 9% Ni steels, austenitic Cr-Mn or Cr-Mn-Ni steels, and austenitic Cr-Ni stainless steels.
Low-alloy steels are typically employed in moderate cryogenic applications down to about -100°C, for manufacturing refrigeration equipment, cryogenic transport vessels, above-ground ethylene storage tanks, and petrochemical processing equipment.
In countries like the USA, UK, and Japan, 9% Ni steel (e.g., ASTM A353) is widely utilized in more demanding low-temperature structures, such as storage and transportation tanks for liquefied natural gas (LNG) at -162°C, liquid oxygen storage at -183°C, and in the fabrication of air separation units for producing liquid oxygen and nitrogen.
Austenitic stainless steels, particularly grades like 304L, 316L, and 347, are excellent cryogenic structural materials, offering exceptional low-temperature toughness, superior weldability, and low thermal conductivity. These steels maintain their ductility down to liquid helium temperatures (-269°C) and are extensively used in extreme low-temperature applications, including liquid hydrogen (-253°C) and liquid oxygen transport and storage tanks, as well as in superconducting magnet cryostats. However, their higher chromium and nickel content makes them more expensive, necessitating careful cost-benefit analysis for each application.
When selecting the welding construction methods and conditions for low-temperature steel, the focus lies on preventing the deterioration of low-temperature toughness at the welding joints and avoiding welding cracks.
There’s no fundamental difference between the groove shapes of welding joints for low-temperature steel, ordinary carbon steel, low-alloy steel, or stainless steel; they can be processed in the standard way. However, for 9Ni steel, the groove angle should ideally be no less than 70 degrees, and the blunt edge should preferably be no less than 3mm.
All low-temperature steel can be cut using an oxyacetylene flame. However, when gas-cutting 9Ni steel, the cutting speed should be slightly slower than when gas-cutting ordinary carbon structural steel. If the steel thickness exceeds 100mm, the cut can be preheated to 150-200℃ before gas-cutting, but it must not exceed 200℃.
Gas cutting does not have any adverse effects on the areas affected by welding heat. However, due to the self-hardening properties of nickel-containing steel, the cut surface will harden.
To ensure satisfactory performance of the welded joint, it’s best to use a grinding wheel to smooth and clean the cut surface before welding.
During welding construction, if it’s necessary to remove the weld bead or base material, one can use arc-air gouging. However, before reworking, the groove surface should still be cleaned and polished.
Oxyacetylene flame gouging should not be used because it risks overheating the steel.
Low-temperature steel can be welded using typical methods such as arc welding, submerged arc welding, and gas metal arc welding.
Arc welding is the most commonly used method for low-temperature steel, and it can be applied in various welding positions. Its heat input is approximately 18~30KJ/cm.
When using low-hydrogen electrodes, completely satisfactory weld joints can be achieved, which not only exhibit good mechanical properties but also excellent notch toughness.
In addition, arc welding has the advantages of simple and inexpensive welding machines, less investment in equipment, and no limitations in position or direction.
The heat input of submerged arc welding for low-temperature steel is around 10~22KJ/cm. It is widely used due to its simplicity, high welding efficiency, and easy operation.
However, due to the insulating effect of the flux, it slows down cooling, leading to a higher tendency to form hot cracks.
In addition, impurities and silicon may enter the weld metal from the flux, which can exacerbate this tendency. Therefore, when using submerged arc welding, the choice of wire and flux should be carefully considered, and operations should be carried out meticulously.
CO2 gas shielded welding produces joints with lower toughness, and thus it is not used in welding low-temperature steel.
Tungsten inert gas (TIG) welding is typically manually operated, and its heat input is limited to the range of 9~15KJ/cm. While the welding joint produced exhibits satisfactory performance, this method is not applicable when the steel thickness exceeds 12mm.
Gas metal arc welding (MIG) is the most widely used automatic or semi-automatic welding method for low-temperature steel, with a heat input of 23~40KJ/cm.
Based on the droplet transfer method, it can be divided into short-circuit transfer (lower heat input), globular transfer (higher heat input), and pulsed-spray transfer (highest heat input). Short-circuit MIG welding might have insufficient fusion depth, which may lead to incomplete fusion defects.
Other MIG welding modes may also have similar issues, but to varying degrees. To achieve a satisfactory fusion depth by making the arc more concentrated, a few to several tens of percent of CO2 or O2 can be introduced into the pure argon used as a shielding gas.
The appropriate percentage should be determined experimentally, based on the specific type of steel being welded.
Welding materials (including electrodes, welding wires, and fluxes), should generally be selected according to the welding method adopted, joint form, groove shape, and other necessary characteristics.
For low-temperature steel, the most important thing is to ensure that the weld metal has low-temperature toughness that matches the parent metal, and to minimize the amount of diffused hydrogen.
(1) Aluminum-Killed Steel
Aluminum-killed steel is highly sensitive to post-weld cooling speed. Electrodes used in manual arc welding for aluminum-killed steel are typically Si-Mn low-hydrogen type or 1.5% Ni type, 2.0% Ni type.
To reduce the heat input of welding, aluminum-killed steel usually adopts multi-layer welding with thin electrodes of 3~3.2mm. This can utilize the secondary heat cycle of the upper welding pass to refine the grains.
The impact toughness of the weld metal welded with Si-Mn electrodes at 50℃ will sharply decrease as the heat input increases. For example, when the heat input increases from 18KJ/cm to 30KJ/cm, the toughness will lose more than 60%. The 1.5% Ni type and 2.5% Ni type electrodes are not sensitive to this, hence they are the best choice for welding.
Submerged arc welding is a common automatic welding method for aluminum-killed steel. The best composition for the welding wire used in submerged arc welding contains 1.5~3.5% nickel and 0.5~1.0% molybdenum.
According to literature, with the use of 2.5%Ni-0.8%Cr-0.5%Mo or 2%Ni welding wire and the appropriate flux, the average toughness value of the weld metal at -55℃ can reach 56-70J (5.7~7.1Kg/fm). Even with 0.5%Mo welding wire and manganese alloy alkaline flux, as long as the heat input is controlled below 26KJ/cm, a weld metal with 55J (5.6Kg/f.m) can still be made.
When choosing flux, pay attention to the matching of Si and Mn in the weld metal. Tests have shown that different Si, Mn content in the weld metal can greatly affect its toughness. The optimal toughness is obtained with 0.1~0.2% Si and 0.7~1.1% Mn. This should be noted when selecting welding wires and fluxes.
Tungsten Inert Gas (TIG) and Metal Inert Gas (MIG) welding are less commonly used in aluminum-killed steel. The aforementioned welding wires for submerged arc welding can also be used for TIG welding.
(2) 2.5Ni Steel and 3.5Ni Steel
For submerged arc welding or MIG welding of 2.5Ni and 3.5Ni steels, welding wires with the same material as the parent metal can generally be used. However, as shown in Wilkinson’s formula, Mn is a hot-cracking inhibitor for low-nickel low-temperature steel.
Keeping the manganese content in the weld metal around 1.2% is beneficial for preventing arc pit cracks and other hot cracks. This should be prioritized when selecting the combination of welding wire and flux.
The temper embrittlement tendency of 3.5Ni steel is high, so after post-weld heat treatment for residual stress relief (e.g., 620℃×1 hour, then furnace cooling), the toughness will dramatically decrease from 3.8Kg/f.m to 2.1Kg/f.m and fail to meet the specification.
The temper embrittlement tendency of the weld metal made by the 4.5%Ni-0.2%Mo welding wire is much smaller, and using this wire can avoid the aforementioned difficulty.
(3) 9Ni Steel
9Ni steel is usually subjected to quenching-tempering or double-normalizing-tempering heat treatment to maximize its low-temperature toughness. However, the weld metal of this steel can’t undergo the aforementioned heat treatment.
Therefore, using ferrite welding materials makes it hard to obtain weld metal with low-temperature toughness comparable to the parent metal. The most commonly used are high-nickel welding materials.
The weld metal of such welding materials is a complete austenitic structure. Despite the drawbacks of lower strength compared to 9Ni steel parent metal and high cost, brittle fracture is no longer a serious problem for it.
From the above, we know that:
Since the weld metal is entirely austenitic, the low-temperature toughness of the weld metal welded with the used electrodes and welding wires can fully compete with the parent metal, although its tensile strength and yield point are lower than the parent metal.
Nickel-containing steel has self-hardening characteristics, so most electrodes and welding wires have taken measures to limit the carbon content to achieve good weldability.
In welding materials, Mo is an important strengthening element, while Nb, Ta, Ti, and W are important toughening elements. Their importance has been fully recognized in the selection and configuration of welding materials.
When the same welding wire is used, the strength and toughness of the submerged arc welding weld metal are somewhat inferior to those of MIG welding. This might be due to the slower cooling speed of the weld and the possible impurities or Si infiltration from the flux.
A333–GR6 steel is a low-temperature steel, with the lowest usage temperature at -70℃, usually delivered in a normalized or normalized plus tempered state. A333-GR6 steel has a low carbon content, thus it has a small hardening and cold cracking tendency, good toughness, and plasticity.
It generally does not easily produce hardening and cracking defects, and has good weldability.
ER80S-Ni1 argon arc welding wire with W707Ni electrodes can be used, applying combined argon-electric welding, or ER80S-Ni1 argon arc welding wire can be used for full argon arc welding to ensure good toughness of the welding joint.
The brand of argon arc welding wire and electrode can be chosen from products with the same performance, but the owner’s approval must be obtained before use.
During welding, for pipes with a diameter smaller than 76.2mm, an I-type butt joint and full argon arc welding are used; for pipes with a diameter larger than 76.2 mm, a V-type groove is opened and the method of argon arc root and multi-layer filling argon-electric welding or full argon arc welding is used.
The specific practices depend on the pipe diameter and wall thickness approved by the owner.
(1) Pre-heating before welding
When the ambient temperature is below 5℃, preheating of the weldment is required.
The preheating temperature is 100~150℃; the preheating range is 100mm on both sides of the weld; oxyacetylene flame (neutral flame) is used for heating, and the temperature is measured 50~100mm away from the center of the weld by a temperature-sensing pen, with evenly distributed temperature points for better temperature control.
(2) Post-weld heat treatment
In order to improve the notch toughness of low-temperature steel, the generally used materials have already undergone quenching and tempering. Improper post-weld heat treatment often deteriorates its low-temperature performance, which should receive sufficient attention.
Therefore, except for conditions where the weldment thickness is larger or the constraint conditions are very strict, low-temperature steel usually does not undergo post-weld heat treatment.
For instance, the welding of the newly added LPG pipeline in CSPC does not require post-weld heat treatment.
If post-weld heat treatment is indeed needed in some projects, the heating rate, constant temperature time, and cooling rate of post-weld heat treatment must be strictly carried out according to the following provisions:
The constant temperature time should be 1h per 25mm wall thickness and not less than 15min. The temperature difference between the highest and lowest temperatures during the constant temperature period should be less than 65℃.
After the constant temperature, the cooling rate should not be greater than 65×25/δ ℃/h, and not more than 260℃/h. Below 400℃, natural cooling is acceptable. Computer-controlled heat treatment equipment should be used.
(1) Strict preheating as per the regulations, controlling the interlayer temperature within 100~200℃. Each weld should be completed in one go, if interrupted, slow cooling measures should be taken.
(2) Arc scratches on the surface of the weldment are strictly forbidden. Upon arc extinguishing, the crater should be filled and any defects ground off with a grinding wheel. The joints between layers in multi-layer welding should be staggered.
(3) Line energy must be strictly controlled, using small currents, low voltages, and quick welding. For W707Ni electrodes with a diameter of 3.2mm, the welding length per electrode must exceed 8cm.
(4) Short-arc, non-swinging operation must be adopted.
(5) Full penetration welding process must be used, and it should strictly follow the requirements in the welding process specifications and welding process card.
(6) The weld reinforcement should be 0~2mm, and the widening of the weld should be ≤2mm on each side.
(7) After the appearance inspection of the weld is passed, non-destructive testing can only be conducted after at least 24 hours. The standard JB 4730-94 should be applied to butt welding seams of the pipe.
(8) The standard “Pressure Vessel: Non-destructive Testing of Pressure Vessels” should be adhered to, and level II qualification should be achieved.
(9) Weld repairs should be carried out before post-weld heat treatment. If repairs are required after heat treatment, the weld must be re-treated with heat after the repair.
(10) If the geometrical size of the weld surface is non-compliant, it is permissible to grind it, provided that the thickness after grinding does not fall below the design requirements.
(11) For general welding defects, a maximum of two repairs is allowed. If it still fails to pass after two repairs, the weld should be cut off and re-welded according to the complete welding process.