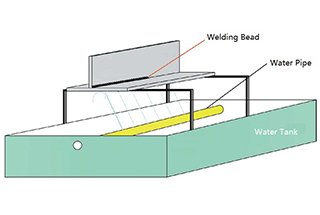
Have you ever wondered how to effectively weld different types of stainless steel? This article dives into the specialized welding methods for martensitic and duplex stainless steel, detailing the challenges and solutions specific to each type. By exploring preheating techniques, material selection, and post-weld treatments, you’ll learn how to achieve strong, durable welds while minimizing risks like cold cracking and embrittlement. This guide provides crucial insights for anyone looking to master the nuances of welding these complex materials.
Martensitic stainless steel is a unique class of ferrous alloys characterized by a martensitic crystalline structure at room temperature. This microstructure results from rapid cooling (quenching) from the austenite phase, creating a hard, metastable structure. The distinguishing feature of martensitic stainless steels is their ability to undergo significant changes in mechanical properties through heat treatment processes.
These alloys are renowned for their hardenability, achieved through a carefully controlled heat treatment cycle of austenitizing, quenching, and tempering. This process allows for tailoring of properties such as hardness, strength, and toughness to meet specific application requirements.
Martensitic stainless steels typically contain 11.5-18% chromium, which provides corrosion resistance, and 0.1-1.2% carbon, which enables the formation of martensite and contributes to hardenability. Some grades may also include small amounts of nickel, molybdenum, or vanadium to enhance specific properties.
Common grades of martensitic stainless steel include:
Related reading: Stainless Steel Grades
Martensitic stainless steel can be welded using various electric arc welding techniques, each offering specific advantages depending on the application and desired outcomes.
Shielded Metal Arc Welding (SMAW), also known as stick welding, remains the primary method due to its versatility and cost-effectiveness. However, advanced processes such as Gas Metal Arc Welding (GMAW) with carbon dioxide shielding gas or Gas Tungsten Arc Welding (GTAW) using argon-carbon dioxide mixed shielding gas have gained prominence in recent years. These methods significantly reduce hydrogen content in the weld pool, thereby minimizing the risk of hydrogen-induced cold cracking in the heat-affected zone (HAZ).
For optimal results, preheat and post-weld heat treatment are often necessary when welding martensitic stainless steels. Preheating to 200-300°C (392-572°F) helps reduce cooling rates and thermal stresses, while post-weld heat treatment at 650-750°C (1202-1382°F) can relieve residual stresses and temper the martensite structure, enhancing the weld’s mechanical properties and corrosion resistance.
When selecting filler metals, it’s crucial to choose compositions that closely match the base metal or provide slightly overmatching strength. Low-hydrogen electrodes (E410 or E410NiMo) are preferred for SMAW, while ER410 or ER410NiMo wires are suitable for GMAW and GTAW processes.
Emerging technologies such as Laser Beam Welding (LBW) and Electron Beam Welding (EBW) are also showing promise for joining martensitic stainless steels, offering high precision and minimal heat input, which can be advantageous for thin sections or heat-sensitive components.
Generally, when higher strength is required in the weld, Cr13 martensitic stainless steel welding rods and wires are utilized to make the chemical composition of the weld metal similar to that of the base metal, but this increases the likelihood of cold cracking.
Considerations:
a. Preheating is necessary prior to welding and the temperature should not exceed 450°C to prevent embrittlement at 475°C.
A post-welding heat treatment must be performed.
Once the temperature has cooled to 150-200°C, a post-welding heat treatment should be carried out for 2 hours to allow for the transformation of all parts of the austenite into martensite, followed by high temperature tempering where the temperature is raised to 730-790°C.
The holding time should be 10 minutes per 1mm of plate thickness, but not less than 2 hours, and finally it should be air cooled.
b. To prevent cracking, the content of S and P in the welding rods and wires should be less than 0.015%, and the Si content should not be greater than 0.3%.
An increase in Si content can cause the formation of coarse primary ferrite, which decreases the plasticity of the joint.
The carbon content should typically be lower than that of the base metal, which can reduce its hardenability.
The weld metal of Cr Ni austenitic steel has a high level of plasticity, which can alleviate the stress produced during the martensitic transformation in the heat-affected zone.
Additionally, the Cr Ni austenitic stainless steel type welds have a high solubility for hydrogen, which can decrease the diffusion of hydrogen from the weld metal to the heat-affected zone and effectively prevent cold cracks, thus preheating is not necessary.
However, the strength of the weld is relatively low and cannot be enhanced through post-weld heat treatment.
Martensitic stainless steel has a high chromium content, which significantly increases its ability to be hardened.
Regardless of its initial state before welding, welding always results in the formation of martensite near the seam.
As the hardening tendency increases, the joint becomes more prone to cold cracking, especially when there is hydrogen present. In such conditions, martensitic stainless steel is also prone to the formation of hazardous hydrogen-induced delayed cracking.
Measures:
Martensitic stainless steels, particularly those with higher levels of ferrite-forming elements, have a higher tendency for grain growth.
A slow cooling rate can lead to the formation of coarse ferrite and carbide in the welding heat-affected zone (HAZ), whereas a rapid cooling rate can cause hardening and the formation of coarse martensite in the HAZ.
These coarse structures reduce the plasticity and toughness of the HAZ of martensitic stainless steel, making it brittle.
Countermeasures:
Preheating prior to welding is a crucial technique to prevent cold cracks and ensure the structural integrity of the weldment. This process reduces the cooling rate in the heat-affected zone (HAZ) and minimizes residual stresses, thereby mitigating the risk of hydrogen-induced cracking.
For steels with carbon content between 0.1% to 0.2%, the recommended preheating temperature typically ranges from 200°C to 260°C (392°F to 500°F). This temperature range is sufficient to drive out moisture and reduce the thermal gradient between the weld and base metal.
High-strength steels, particularly those with yield strengths exceeding 690 MPa (100 ksi), often require higher preheating temperatures. For these materials, preheating to temperatures between 400°C to 450°C (752°F to 842°F) is common. This elevated temperature range helps to further reduce cooling rates and manage the formation of hard, brittle microstructures in the HAZ.
It’s important to note that the optimal preheating temperature depends not only on carbon content but also on other alloying elements, section thickness, ambient temperature, and the specific welding process being used. Welding engineers should consult relevant standards (such as AWS D1.1 or ISO 13916) and perform necessary calculations (e.g., carbon equivalent) to determine the most appropriate preheating regime for each application.
Post-weld cooling is a critical step in the welding process, particularly for high-strength steels and thick sections. The weldment should not be directly heated from the welding temperature to the tempering temperature, as the austenite formed during welding may not have fully transformed.
Immediate heating and tempering after welding can lead to several detrimental effects:
These microstructural changes significantly reduce the toughness and overall mechanical properties of the weldment.
To mitigate these issues, a controlled cooling process is essential:
1. For low-strength weldments:
2. For high-strength and thick weldments:
This controlled cooling process ensures that:
The specific cooling rate and intermediate holding temperatures may vary depending on the material composition, section thickness, and desired mechanical properties. It’s crucial to follow the welding procedure specification (WPS) or consult with metallurgical experts for optimal results.
In some cases, advanced cooling techniques such as forced air cooling or even quenching may be employed, but these must be carefully controlled to avoid introducing new issues such as distortion or cracking.
Post-weld heat treatment (PWHT) is a critical process designed to optimize the mechanical properties and structural integrity of welded components. Its primary objectives are to reduce hardness in the weld and heat-affected zone (HAZ), enhance ductility and toughness, and mitigate welding residual stresses.
PWHT typically involves two main processes: tempering and full annealing. For tempering, the recommended temperature range is 650-750°C (1202-1382°F). The component should be held at this temperature for approximately 1 hour, followed by controlled air cooling. This process effectively relieves internal stresses while maintaining a balance between strength and ductility.
In cases where post-weld machining is required, full annealing can be employed to achieve minimum hardness and maximum machinability. The annealing process involves heating the weldment to a temperature range of 830-880°C (1526-1616°F) and maintaining this temperature for 2 hours. Subsequently, the component undergoes slow furnace cooling to 595°C (1103°F), followed by air cooling to room temperature. This controlled cooling rate is crucial for achieving the desired microstructure and properties.
It’s important to note that the specific PWHT parameters may vary depending on factors such as material composition, section thickness, and service requirements. For critical applications, it’s advisable to consult relevant industry standards (e.g., ASME BPVC Section IX) and perform mechanical testing to validate the effectiveness of the heat treatment process.
The choice of welding electrodes for martensitic stainless steel is critical and falls into two main categories: chromium stainless steel electrodes and chromium-nickel austenitic stainless steel electrodes. This selection significantly impacts the weld’s mechanical properties, corrosion resistance, and overall integrity.
Chromium stainless steel electrodes, such as E410-15 (AWS A5.4) or E410-16, are commonly used. These electrodes, corresponding to the Chinese standards E1-13-15 (G207) and E1-13-16 (G202) respectively, offer good strength and moderate corrosion resistance. They are particularly suitable when matching the base metal composition is desired.
For applications requiring enhanced ductility and corrosion resistance, chromium-nickel austenitic stainless steel electrodes are preferred. Popular choices include:
The “L” grade electrodes (e.g., 308L, 316L) have lower carbon content, reducing the risk of sensitization and improving resistance to intergranular corrosion in high-temperature service.
Selection between these electrodes depends on factors such as:
Duplex stainless steel has both the advantages and disadvantages of austenitic and ferritic steel, and reduces their respective weaknesses.
(1) The risk of hot cracking is much lower compared to austenitic steel.
(2) The risk of cold cracking is significantly lower compared to ordinary low alloy high strength steel.
(3) After cooling in the heat-affected zone, a higher amount of ferrite is retained, increasing the risk of corrosion and hydrogen-induced cracking (embrittlement).
(4) The welded joint of duplex stainless steel is prone to the precipitation of δ phase, an intermetallic compound of Cr and Fe.
Its formation temperature ranges from 600°C to 1000°C and can vary based on the specific steel grade.
Table 1 Temperature Range of Solution Treatment, Phase δ and 475 ℃ Brittleness of Duplex Stainless Steel
Content | 2205 dual phase steel and 2507, etc | Super duplex steel 00Cr25Ni7Mo3CuN |
Solid solution temperature/℃ | 1040 | 1025~1100 |
Peeling temperature when heated in air/℃ | 1000 | 1000 |
Phase δ formation temperature/℃ | 600~1000 | 600~1000 |
475 ° C embrittlement temperature/℃ | 300~525 | 300~525 |
The welding process for duplex stainless steel involves TIG welding first, followed by electrode arc welding.
When using submerged arc welding, the heat input and interpass temperature must be closely monitored and excessive dilution must be avoided.
Note:
When TIG welding is used, 1-2% nitrogen should be added to the shielding gas (adding more than 2% nitrogen may increase porosity and cause instability in the arc). The addition of nitrogen helps absorb nitrogen from the weld metal, preventing nitrogen loss through diffusion in the weld surface area, and contributes to stabilizing the austenite phase in the welded joint.
Welding materials with higher levels of austenite-forming elements (such as Ni, N) are chosen to encourage the transformation of ferrite in the weld into austenite.
The 22.8.3L electrode or welding wire is commonly used for welding 2205 steel, while the 25.10.4L electrode or 25.10.4R electrode is frequently used for welding 2507 steel.
Table 2 Welding materials and FN of Typical duplex stainless steel
Base metal | Welding Material | Chemical composition | Name | FN(%) | ||||||||
C | Si | Mn | Cr | Ni | Mo | N | Cu | W | ||||
2507 | Welding wire | 0.02 | 0.3 | 0.5 | 25 | 10 | 4 | 0.25 | – | – | 2507/P100 | 40~100 |
0.02 | 25 | 10 | 4 | 0.25 | – | – | Sandivick 25.10.4L | |||||
Welding core | 0.03 | 0.5 | 1 | 25 | 9.5 | 3.6 | 0.22 | – | – | Avesta 2507/p100 | ||
0.04 | 25 | 10.5 | 4 | 0.25 | – | – | Sandivick 25.10.4L | |||||
Zeron100 | Welding wireWelding core | 0.04 | 1.2 | 2.5 | 25 | 10 | 4 | 0.22 | 1 | 1 | 22.9.4CuWL 22.9.4CuWLB | 40~60 |
2205 | Welding wire | 0.02 | 0.5 | 1.6 | 22.5 | 8 | 3 | 0.14 | – | – | Sandivick 22.8.3L | 40~60 |
Welding core | 0.03 | 1.0 | 0.8 | 22.5 | 9.5 | 3 | 0.14 | – | – | Sandivick 22.8.3R |
(1) During the welding process, the control of welding energy, interpass temperature, preheating, and material thickness will affect the cooling rate and subsequently affect the structure and properties of the weld and heat-affected zone.
To achieve optimal weld metal properties, it is recommended to control the maximum interpass temperature at 100°C. If post-weld heat treatment is necessary, the interpass temperature restrictions may be lifted.
(2) It is preferred to avoid post-weld heat treatment for duplex stainless steel.
If post-weld heat treatment is necessary, water quenching is the method used. During heat treatment, the heating should be rapid, and the holding time at the heat treatment temperature should be between 5-30 minutes, sufficient to restore the phase balance.
Oxidation of metal is a concern during heat treatment, so the use of an inert gas for protection should be considered.