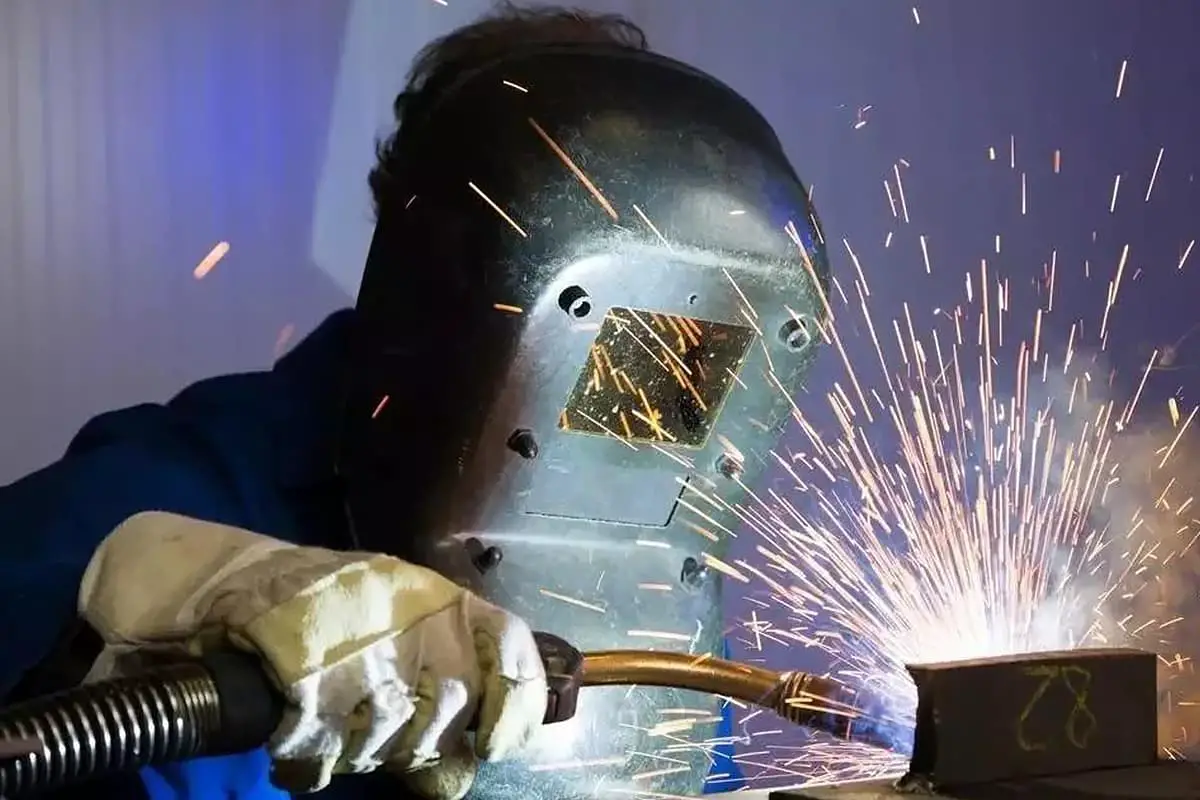
Welding is the backbone of modern manufacturing, but with so many methods available, how do you choose the right one for your project? In this blog post, we’ll dive into the world of welding and explore the most common techniques used in the industry today. Our expert mechanical engineer will guide you through the pros and cons of each method, helping you make an informed decision for your next project. Get ready to spark your knowledge and take your welding skills to the next level!
Manual arc welding is the earliest developed and still the most widely used welding method among all arc welding techniques.
It employs an externally coated welding rod as both the electrode and filler metal, with the arc burning between the end of the welding rod and the surface of the workpiece being welded.
Under the thermal effects of an electric arc, the coating can generate gas to protect the arc on one hand, and on the other, it can produce slag to cover the surface of the weld pool, preventing the molten metal from interacting with the surrounding gas.
The more significant role of the slag is to undergo physiochemical reactions with the molten metal or introduce alloying elements, thus enhancing the properties of the weld seam.
Arc welding equipment is simple, portable, and flexible in operation. It can be used for welding short seams in repair and assembly, especially for welding in hard-to-reach areas.
With the appropriate welding rod, arc welding can be applied to most industrial carbon steel, stainless steel, cast iron, copper, aluminum, nickel, and their alloys.
This is a type of non-consumable electrode gas shielded arc welding, where an arc between the tungsten electrode and the workpiece causes the metal to melt and form a weld seam.
During the welding process, the tungsten electrode does not melt and only serves as an electrode.
Simultaneously, argon or helium gas is fed from the welding torch nozzle for protection. Additional metal can be added as needed, a process internationally known as TIG welding.
Tungsten Inert Gas (TIG) welding is an excellent method for joining thin sheet metal and for root pass welding due to its superior control over heat input.
This method can be applied to almost all metal connections, especially useful for welding metals like aluminum and magnesium, which form refractory oxides, as well as reactive metals like titanium and zirconium.
While this welding method offers high-quality welds, its speed is slower compared to other arc welding techniques.
This welding method uses the heat from the burning arc between the continuously fed welding wire and the workpiece. The arc is shielded by gas sprayed from a torch nozzle.
Gas Metal Arc Welding typically uses shielding gases such as argon, helium, CO2, or a mixture of these gases.
When argon or helium is used as the shielding gas, it’s referred to as Metal Inert Gas Welding (MIG), a term commonly used internationally.
When a mixture of inert gas and oxidizing gas (O2, CO2) is used as the shielding gas, or when CO2 gas or a mixture of CO2 + O2 is used, it is universally referred to as Metal Active Gas (MAG) welding.
The primary advantages of MAG welding include the ability to conveniently weld in a variety of positions, coupled with high welding speed and deposition rate.
MAG welding is compatible with most major metals, including carbon steel and alloy steel. In contrast, Gas Metal Arc Welding (GMAW) with an inert gas shield is suitable for stainless steel, aluminum, magnesium, copper, titanium, zirconium, and nickel alloys. This welding method can also be used for spot welding.
Plasma arc welding is a type of non-consumable electrode arc welding. It utilizes a compressed arc between the electrode and the workpiece (known as a transferred arc) to achieve welding.
The electrode typically used is made of tungsten. The plasma gas that generates the plasma arc can be argon, nitrogen, helium, or a mixture of two.
Additionally, an inert gas is used for protection through the nozzle. During welding, filler metal can be added, although it’s not always necessary.
During plasma arc welding, due to its straight arc and high energy density, the arc penetration is strong. The keyhole effect produced during plasma arc welding allows for butt welding of most metals within a certain thickness range without the need for a groove, ensuring consistent melt-through and even weld seams.
Therefore, plasma arc welding has a high productivity rate and excellent weld quality. However, plasma arc welding equipment, including the nozzle, is relatively complex and requires high control over welding process parameters.
Most metals that can be welded with tungsten inert gas (TIG) welding can also be welded using plasma arc welding.
Compared to this, plasma arc welding can be more effectively performed for extremely thin metals of less than 1mm.
Tubular wire arc welding also uses the burning arc between the continuously fed welding wire and the workpiece as the heat source for welding, which can be considered as a type of gas metal arc welding. The welding wire used is tubular, filled with various components of flux.
During welding, protective gas, primarily CO2, is added externally. The flux, when heated, decomposes or melts, thereby providing slag to protect the weld pool, alloy diffusion, and arc stabilization.
Flux-cored arc welding, beyond the benefits of aforementioned gas metal arc welding, is metallurgically superior due to the function of the internal flux. This method can be applied to weld various joints of most ferrous metals.
Flux-cored arc welding has been widely adopted in several advanced industrial countries. The term “flux-cored wire” is what we currently refer to as “tubular welding wire.”
This category of welding methods uses resistance heat as the energy source, including electric slag welding powered by molten slag resistance heat and resistance welding powered by solid resistance heat. Electric slag welding, having unique features, will be discussed later.
This section primarily introduces various types of resistance welding that use solid resistance heat as the energy source, including spot welding, seam welding, projection welding, and butt welding.
Resistance welding is a method that melts the contact surfaces between two workpieces by using the resistive heat generated when current passes through the workpieces under a certain electrode pressure. This process usually employs a large current.
To prevent arcing at the contact surface and to forge-weld the seam metal, pressure must be applied consistently during welding. In this type of resistance welding, the cleanliness of the workpiece surface is paramount for achieving stable weld quality.
Therefore, it is essential to clean the contact surfaces between the electrode and workpiece, and between the workpieces prior to welding.
Spot welding, seam welding, and projection welding are characterized by high welding current (single-phase, from a few thousand to tens of thousands of amperes), short energizing time (from a few cycles to a few seconds), expensive and complex equipment, and high productivity, making them suitable for mass production.
These methods are primarily used for welding thin sheet assemblies less than 3mm in thickness. They can weld all kinds of steels, non-ferrous metals like aluminum and magnesium, their alloys, and stainless steel.
Electron beam welding is a method that uses the thermal energy produced when a concentrated high-speed electron beam strikes the surface of a workpiece.
During electron beam welding, an electron gun generates and accelerates the electron beam.
Common types of electron beam welding include: high vacuum electron beam welding, low vacuum electron beam welding, and non-vacuum electron beam welding.
The first two methods are performed inside a vacuum chamber. The preparation time for welding (primarily the time for vacuum pumping) is quite extensive, and the size of the workpiece is limited by the size of the vacuum chamber.
Compared to arc welding, electron beam welding is distinguished by its deep weld penetration, narrow melt width, and high metallic purity. It’s versatile, capable of precision welding on thin materials, as well as handling very thick components, up to 300mm.
All metals and alloys that can be fusion-welded using other methods are suitable for electron beam welding. It’s primarily used for high-quality product welding.
Moreover, it can solve the welding problems associated with dissimilar metals, easily oxidized metals, and difficult-to-melt metals. However, it is not suitable for mass-produced items.
Laser welding utilizes a high-powered, coherent, monochromatic stream of photons, focused into a laser beam, as the heat source for the welding process. This welding approach typically involves continuous power laser welding and pulsed power laser welding.
The advantage of laser welding is that it does not need to be conducted in a vacuum, but its downside is that its penetration power is not as strong as electron beam welding.
Laser welding allows for precise energy control, thereby enabling the welding of precision micro-devices. It can be applied to many metals, especially solving the welding of some difficult-to-weld and dissimilar metals.
The energy for brazing can come from either chemical reaction heat or indirect thermal energy. It employs a metal with a lower melting point than the material being brazed as the filler.
This metal melts upon heating, and capillary action draws the filler into the gap on the contact surface of the joint, wetting the surface of the metal being brazed.
This process results in a brazed joint through mutual diffusion between the liquid and solid phases. Therefore, brazing is a welding method that involves both solid and liquid phases.
Brazing operates at a relatively low heating temperature, leaving the base metal un-melted without requiring any applied pressure.
However, it’s necessary to take certain measures to clean the surface of the workpiece from oil, dust, and oxidation layers prior to brazing. This is a crucial step in ensuring good wetting of the workpiece and the quality of the joint.
Brazing is classified as hard brazing when the liquidus line of the braze alloy is above 450℃, but below the melting point of the base metal. When it falls below 450℃, it’s termed as soft brazing.
Depending on the heat source or heating method, brazing can be categorized into flame brazing, induction brazing, furnace brazing, dip brazing, resistance brazing, and more.
Given the relatively low heating temperature during brazing, there’s minimal impact on the material properties of the workpiece, with reduced stress deformation. However, the strength of brazed joints tends to be lower, with poor heat resistance.
Brazing can be used for joining carbon steel, stainless steel, high-temperature alloys, aluminum, copper, and other metal materials. It also allows for the connection of dissimilar metals, as well as metals and non-metals.
It’s particularly suitable for joints that bear low loads or operate at room temperature, and especially applicable for precision, miniature, and complex multi-brazed seam workpieces.
Electroslag welding is a method that utilizes the resistive heat of molten slag as an energy source. The welding process is conducted in an assembly gap formed by the end faces of two workpieces and two water-cooled copper sliders in a vertical welding position.
During welding, the resistive heat generated by the electric current passing through the molten slag is used to melt the ends of the workpieces.
Depending on the shape of the electrode used during welding, electroslag welding can be categorized into wire electrode electroslag welding, plate electrode electroslag welding, and consumable nozzle electroslag welding.
The advantages of electroslag welding include its ability to weld large workpiece thicknesses (ranging from 30mm to over 1000mm) and its high production rate. It is predominantly employed for the welding of butt joints and T-joints.
Electroslag welding can be utilized in the welding of various steel structures, and also in the assembly welding of castings.
Due to the slow heating and cooling process, the electroslag welding joint has a wide heat-affected zone with coarse microstructures, resulting in improved toughness. Therefore, it generally requires post-weld heat treatment.
High-frequency welding employs solid-state resistance heat as its energy source.
During the welding process, the high-frequency current generates resistance heat within the workpiece, heating the surface of the welding area to a molten or near-plastic state.
Subsequently, a forging force is applied (or not applied), resulting in the fusion of the metals. Thus, it is a type of solid-state resistance welding method.
High frequency welding can be categorized into contact high frequency welding and induction high frequency welding based on how the high-frequency current generates heat in the workpiece.
In contact high frequency welding, the high frequency current is transferred into the workpiece through mechanical contact. In induction high frequency welding, the high frequency current induces an electric current within the workpiece via the coupling effect of an external induction coil.
High frequency welding is a highly specialized welding method that requires dedicated equipment according to the product.
It offers a high production rate, with welding speeds up to 30m/min. It is primarily used for welding longitudinal or spiral seams when manufacturing tubes.
Gas welding is a type of welding method that uses a gas flame as the heat source. The most commonly used is the oxy-acetylene flame, with acetylene as the fuel.
Although the equipment is simple and easy to use, gas welding has a slower heating rate and lower productivity. It also produces a larger heat-affected zone and is likely to result in significant deformation.
Gas welding can be used for the joining of many ferrous metals, non-ferrous metals, and their alloys. It is typically utilized for repair and single-piece thin sheet welding.
Pressure gas welding, like gas welding, uses a gas flame as the heat source. During the process, the ends of the two workpieces to be joined are heated to a certain temperature, then sufficient pressure is applied to achieve a robust joint.
This method is a type of solid-phase welding. During pressure gas welding, no filler metal is added. It is commonly used for rail welding and rebar welding.
Explosive welding is another solid-state welding method that utilizes the heat from a chemical reaction as its energy source.
However, it leverages the energy generated from an explosive detonation to facilitate the joining of metals. Under the influence of an explosive wave, two pieces of metal can be accelerated and impacted to form a metallic bond in less than a second.
Of all welding methods, explosive welding provides the broadest range for joining dissimilar metals. It can fuse two metallurgically incompatible metals into various transitional joints.
Explosive welding is commonly used for surface cladding of large flat plates and is an efficient method for manufacturing composite plates.
Friction welding is a solid-state welding process powered by mechanical energy. It utilizes the heat generated from mechanical friction between two surfaces to achieve metal connection.
The heat in friction welding is concentrated at the joint, thus the heat-affected zone is narrow.
Pressure must be applied between the two surfaces, and in most cases, the pressure is increased at the end of the heating phase, causing the heated metal to undergo upset forging and bind together. Typically, the joint surface does not melt.
Friction welding offers high productivity, and in theory, virtually all metals that can be hot-forged can be friction-welded. This technique can also be used for welding dissimilar metals.
It is applicable to workpieces with a maximum circular cross-section diameter of 100mm.
Ultrasonic welding is a solid-state welding method that relies on mechanical energy as its power source.
During the process, the workpiece under relatively low static pressure is subjected to high-frequency vibrations produced by the acoustic pole. This induces intense friction at the joint surface, heating it to the welding temperature and forming a bond.
Ultrasonic welding can be used for the joining of most metallic materials, facilitating the welding of metals, dissimilar metals, and the junction between metals and non-metals.
This method is suitable for the repetitive production of metal wires, foils, or thin metal sheets less than 2-3mm thick.
Diffusion welding typically utilizes indirect heat as the source of energy for solid-phase welding. It is usually performed under vacuum or in a protective atmosphere.
During the welding process, the surfaces of the two workpieces to be welded are brought into contact under high temperatures and substantial pressure, and are held there for a certain amount of time to achieve interatomic distances. The subsequent atomic diffusion results in bonding.
Prior to welding, not only does the workpiece surface need to be cleaned of oxides and other impurities, but the surface roughness must also be below a certain value to ensure the quality of the weld.
Diffusion welding exhibits virtually no detrimental effects on the properties of the materials being joined.
It can be used to weld a wide range of both homogenous and heterogeneous metals, as well as some non-metallic materials like ceramics.
Additionally, diffusion welding is capable of joining complex structures and components with significant differences in thickness.