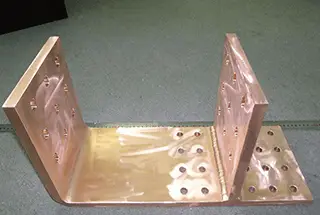
Welding copper and its alloys is a unique challenge due to their high thermal conductivity and tendency to crack. This article covers various welding techniques, materials, and preparation methods essential for achieving successful welds in copper and its alloys. Readers will learn about the specific weldability issues, pre-welding preparations, and the selection of appropriate welding methods and materials. By understanding these factors, one can enhance the performance and reliability of copper welds, crucial for applications in numerous industries.
Copper and copper alloys are extensively utilized across industries due to their exceptional combination of properties. These materials exhibit superior electrical and thermal conductivity, high resistance to oxidation, and excellent corrosion resistance in diverse environments including freshwater, saltwater, alkaline solutions, and organic chemicals. However, it’s important to note their vulnerability to corrosion in oxidizing acids.
Copper alloys demonstrate excellent formability in both cold and hot working processes, coupled with enhanced strength compared to pure copper. Their versatility has led to widespread adoption in critical sectors such as electrical and electronics, chemical processing, food production, power generation, transportation, aerospace, and defense industries.
The industrial production of copper and copper alloys yields a diverse range of materials, typically classified based on chemical composition. The primary categories include:
1. Pure Copper: Soft annealed pure copper is preferred for welding structures. Common grades include T1, T2, T3, T4, and oxygen-free copper variants like TU1 and TU2.
2. Brass: These copper-zinc alloys offer a balance of strength, ductility, and corrosion resistance. Widely used grades include H62, H68, H96, as well as specialized alloys like lead brass (HPb59-1) and tin brass (HSn62-1).
3. Bronze: Originally referring to copper-tin alloys, this term now encompasses copper alloys where zinc or nickel is not the primary alloying element. Notable types include:
4. White Copper (Cupronickel): These copper-nickel alloys exhibit remarkable corrosion resistance, particularly in marine environments.
Each of these copper-based materials offers unique property combinations, allowing engineers and designers to select the optimal alloy for specific applications. The continuous development of new copper alloys and processing techniques continues to expand their potential uses in advanced technologies and demanding environments.
The weldability of copper and copper alloys is comparatively poor, making it much more challenging to weld compared to low carbon steel. The main difficulties are observed in the following aspects:
(1) Poor weld formation capability:
When welding copper and most copper alloys, it is prone to difficulties in achieving fusion, incomplete penetration of the joint, and poor surface formation. This is mainly due to the high thermal conductivity of copper, with thermal conductivity of copper and most copper alloys being 7 to 11 times greater than that of ordinary carbon steel.
As a result, heat is rapidly dissipated from the welding zone. The thicker the workpiece, the more severe the heat dissipation. Although copper has a lower melting point and specific heat capacity compared to iron, it is still challenging to reach the melting temperature in the welding zone, making it difficult for the base metal and filler metal to fuse.
Additionally, the excellent thermal conductivity of copper leads to a broader heat-affected zone, which can result in significant deformation when the workpiece has low rigidity. Conversely, when the rigidity is high, it can cause substantial welding stress within the workpiece.
Poor surface formation in copper and copper alloys is mainly attributed to the fact that the surface tension during melting is one-third that of steel, and the fluidity is 1 to 1.5 times greater than that of steel, making it more susceptible to metal loss during melting.
Therefore, when welding pure copper and most highly conductive copper alloys, in addition to using high-power and high-energy density welding methods, it is also necessary to incorporate varying degrees of preheating. It is not permissible to use single-sided welding without support, and when performing single-sided welding, a backing plate must be added to control the formation of the weld joint.
(2) High susceptibility to heat cracking in welds and heat-affected zones:
The tendency for heat cracking in welds is related to the influence of impurities in the weld and is also influenced by the stresses generated during the welding process. Oxygen is a common impurity found in copper, and it has a significant impact on the tendency for heat cracking in welds.
At high temperatures, copper reacts with oxygen in the air to form Cu2O. Cu2O is soluble in liquid copper but not in solid copper, forming a low-melting-point eutectic. Impurities such as Bi and Pb in copper and copper alloys have low melting points.
During the solidification process of the weld pool, they form low-melting-point eutectics that distribute between dendrites or at grain boundaries, causing significant thermal brittleness in copper and copper alloys. When the weld is in the solid-liquid phase, the low-melting-point eutectics in the heat-affected zone re-melt under the influence of welding stresses, resulting in heat cracks.
Copper and copper alloys have relatively high linear expansion coefficients and shrinkage rates, and they also exhibit strong thermal conductivity. When welding, high-power heat sources are required, resulting in a wider heat-affected zone. As a result, the welded joints experience significant internal stresses, which is another factor that leads to cracking in copper and copper alloy welds.
Furthermore, when welding pure copper, the weld metal consists of a single-phase structure. Due to the high thermal conductivity of pure copper, the weld tends to form coarse grains. This further exacerbates the formation of heat cracks.
Therefore, to prevent the formation of heat cracks when using fusion welding to weld copper and copper alloys, the following metallurgical measures should be taken:
1) Strictly control the content of impurities (such as oxygen, bismuth, lead, sulfur, etc.) in copper.
2) Enhance the deoxidation capability of the weld by adding alloying elements such as silicon, manganese, phosphorus, etc., to the welding wire.
3) Select welding materials that can obtain a duplex structure, which disrupts the continuity of low-melting-point eutectic films and changes the direction of columnar grains.
4) Implement measures such as preheating and slow cooling to reduce welding stresses, minimize root gap size, and increase root pass dimensions to prevent crack formation.
(3) Susceptibility to porosity formation:
When fusion welding copper and copper alloys, the tendency for the formation of porosity is much more significant compared to low carbon steel. To reduce and eliminate porosity in copper welds, the main measures are to reduce the sources of hydrogen and oxygen and to preheat to prolong the existence time of the molten pool, making it easier for gases to escape.
Using welding wires with strong deoxidizers such as aluminum, titanium, etc. (which can also remove nitrogen and hydrogen) or adding elements like aluminum and tin to copper alloys can yield good results in terms of deoxidation.
(4) Decreased welding joint performance:
During the fusion welding process of copper and copper alloys, the weld joints experience severe grain growth, evaporation and burn-off of alloying elements, as well as infiltration of impurities, leading to a decrease in mechanical properties, electrical conductivity, and corrosion resistance of the welded joints.
1) Significant decrease in ductility:
The weld and heat-affected zone experience grain coarsening, and various brittle low-melting-point eutectics appear at grain boundaries, weakening the metal’s bonding strength and significantly reducing the ductility and toughness of the joint. For example, when using pure copper welding electrodes for arc welding or submerged arc welding, the elongation of the joint is only about 20% to 50% of the base material.
2) Decrease in electrical conductivity:
The addition of any element to copper will decrease its electrical conductivity. Therefore, the melting of impurities and alloying elements during the welding process will to some extent deteriorate the electrical conductivity of copper joints.
3) Decreased corrosion resistance:
The corrosion resistance of copper alloys is achieved through alloying with elements such as zinc, manganese, nickel, aluminum, etc. The evaporation and oxidation of these elements during the fusion welding process will to some extent reduce the corrosion resistance of the joint. The generation of welding stresses also increases the risk of stress corrosion.
Measures to improve joint performance mainly involve controlling the content of impurities, reducing alloy burn-off, and performing heat treatment to modify the microstructure of the weld. Minimizing the heat input during welding and applying stress relief treatment after welding are also beneficial.
The welding of copper and copper alloys presents unique challenges due to the material’s exceptional thermal conductivity. A wide array of welding techniques are available, each with specific advantages for different applications. Common methods include gas welding, shielded metal arc welding (SMAW), tungsten inert gas (TIG) welding, gas metal arc welding (GMAW/MIG), and submerged arc welding (SAW).
Selection of the optimal welding method should be based on multiple factors:
Copper’s superior thermal conductivity (nearly 6 times that of steel) necessitates welding methods with high power density and concentrated heat input. This helps overcome rapid heat dissipation and ensures proper fusion. Techniques that offer higher thermal efficiency and focused energy deposition are generally preferred.
The thickness of the copper material significantly influences the choice of welding method:
Emerging technologies like laser welding and hybrid laser-arc welding are gaining traction for copper welding, offering high precision and minimal heat-affected zones.
Proper selection of filler metals, shielding gases, and pre/post-weld heat treatments are crucial for achieving optimal weld quality in copper and its alloys. Additionally, stringent cleanliness and surface preparation are essential due to copper’s sensitivity to oxidation and contamination.
1) Welding Wire:
When selecting welding wire for copper and copper alloys, it’s crucial to not only meet general process and metallurgical requirements but also to carefully control impurity content and enhance deoxidation capabilities. This is essential to prevent the formation of heat cracks and porosity, which are common issues in copper welding.
For pure copper welding, the wire is typically alloyed with deoxidizing elements such as Silicon (Si), Manganese (Mn), and Phosphorus (P). These elements help to scavenge oxygen from the weld pool, reducing the risk of porosity and improving overall weld quality. A widely used option is the high-purity copper welding wire HSCu. This wire is versatile and can be employed in various welding processes:
2) Welding Electrodes:
Arc welding electrodes for copper applications can be categorized into two main types: copper and bronze. Among these, bronze electrodes are more frequently utilized due to their superior performance characteristics.
Copper electrodes, particularly those containing zinc (as in brass alloys), are seldom used in arc welding processes. This is primarily due to the high vapor pressure of zinc at welding temperatures, which can lead to excessive fume generation, porosity, and inconsistent arc behavior.
Bronze electrodes, on the other hand, offer a more stable arc and better weldability. They are particularly effective when welding copper to itself or to other copper alloys. Two commonly used electrode types in this category are:
When selecting electrodes, factors such as the base metal composition, required mechanical properties, and specific application requirements should be carefully considered to ensure optimal welding results.
Pre-treatment requirements for copper and copper alloy weldments are stringent due to the materials’ high thermal conductivity and susceptibility to oxidation. The primary focus of pre-weld cleaning is the removal of contaminants and oxide films to ensure optimal weld quality and performance.
Begin by thoroughly degreasing the weld joint and surrounding area (approximately 30mm on each side) using a suitable solvent such as acetone or isopropyl alcohol. This step is crucial for removing any oils or organic contaminants that could compromise weld integrity.
Following degreasing, employ a two-step chemical cleaning process:
For mechanical oxide removal, utilize a stainless steel wire brush or wheel specifically designated for copper. Pneumatic tools can increase efficiency, but care must be taken to avoid excessive material removal or surface contamination. Continue brushing until a uniform, bright metallic luster is achieved.
Filler metals require similar attention. Clean welding wires mechanically using a lint-free cloth or fine abrasive paper to remove surface oxides immediately before use. For larger operations, consider automated wire cleaning systems to maintain consistent quality.
Post-cleaning, minimize the time between preparation and welding to prevent re-oxidation. If immediate welding is not possible, store prepared components in a controlled environment with low humidity and protect surfaces with suitable anti-oxidation compounds compatible with the welding process.
Implement proper safety measures when handling chemicals, including appropriate personal protective equipment (PPE) and adequate ventilation. Adhere to local environmental regulations for the disposal of spent cleaning solutions.
Gas Welding:
Gas welding is suitable for joining thin copper components, repairing copper parts, or welding non-critical structures. Its versatility makes it particularly useful in maintenance and repair operations.
1) Preheating before welding:
Preheating is essential for gas welding pure copper to mitigate internal stresses, prevent cracking, reduce porosity, and ensure complete penetration. For thin sheets and small weldments, preheat to 400-500°C (752-932°F). Increase the preheating temperature to 600-700°C (1112-1292°F) for thick and large weldments. Brass and bronze alloys typically require slightly lower preheating temperatures due to their different thermal properties.
2) Selection of welding parameters and technique:
Given copper’s high thermal conductivity, the flame energy for welding should be 1-2 times higher than that used for carbon steel. When welding pure copper, it is crucial to maintain a neutral flame.
An oxidizing flame can lead to weld oxidation and loss of alloying elements, compromising joint integrity. Conversely, a carburizing flame increases hydrogen content in the weld, promoting porosity formation.
For thin sheets, employ the left-hand welding technique to minimize grain growth. For workpieces thicker than 6mm (0.24 inches), the right-hand welding method is preferred, as it facilitates higher base metal heating and provides better molten pool visibility, enhancing operational efficiency.
Maintain a swift, continuous movement of the welding torch, avoiding random interruptions in each weld seam. Ideally, complete each weld seam in a single pass to ensure uniformity and reduce the risk of defects.
When welding long seams, incorporate appropriate allowances for shrinkage before welding and ensure proper positioning. Utilize the segmented back-stepping method during welding to minimize deformation and residual stresses.
For stress-bearing or critical copper weldments, implement post-weld treatments:
These post-weld treatments help refine grain structure, relieve residual stresses, and improve overall weld quality, ensuring optimal performance of the welded copper components.
There is an electrode water jacket, made of deoxidized copper TU1. The electrode joint is welded using MIG welding, and the specific welding process is shown in Table 5-37.
Table 5-37 Welding Process Card for TU1 Joint
Welding Process Card for Joint Welding | Number | |||
Joint Diagram:![]() | Base Material Material | TU1 | TU1 | |
Base Material Thickness | 15mm | 15mm | ||
Welding Position | Flat Weld | |||
Welding Technique | Straight Weld Path | |||
Preheating Temperature | 500℃ | |||
Interpass Temperature | ≥500℃ | |||
Nozzle Diameter | Φ26mm | |||
Protective Gas | Ar | Gas Flow Rate (L/min) | Front: 25~30 Back: |
Welding Sequence | |
1 | Inspect the groove dimensions and surface quality. |
2 | Remove any oil or dirt from the groove and its vicinity. Clean the grease by using a 10% NaOH water solution at a temperature of 30~40℃, then rinse with clean water and dry. Remove the oxide film by grinding with a stainless steel wire wheel, then rinse with alkaline water, followed by rinsing with clean water and drying. |
3 | Perform tack welding for the first layer using an outer positioning welding technique. The length should be 100mm, and the distance between weld points should not exceed 300mm. If cracks appear in the tack weld seam, remove them and re-weld. |
4 | Splice the electrodes on a specially designed fixture. Preheat the workpiece using electric heating, with a preheating temperature of 500℃, and ensure that the interlayer temperature remains no lower than 500℃. |
5 | Start welding from the outside to avoid the formation of weld beads on the inside of the weld seam. Ensure the roundness of the electrode inner circle and the smoothness of the inner surface. |
6 | Perform visual inspection. |
7 | Straighten if necessary. |
8 | Perform post-weld heat treatment. |
Welding Specification Parameters
Passes | Welding Method | Welding Material Grade | Welding Material Specification | Types of Current and Polarity | Welding Current (Ampere) | Arc Voltage (Volt) | Welding Speed (mm/per pass) | Remarks |
1~2 | MIG (Semi-Automatic) | HSCu | 1.6 | DCEP | 350~400 | 30~35 | 250~300 |