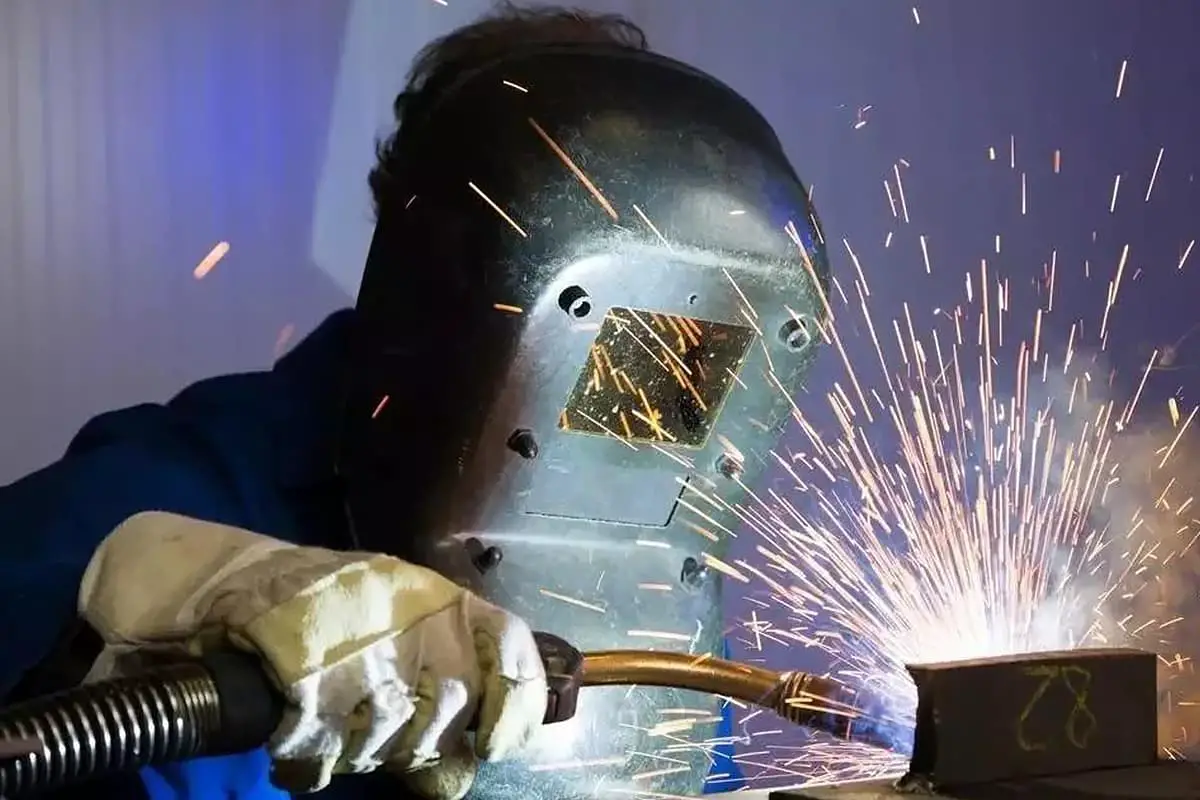
Have you ever wondered why some welded structures fail unexpectedly? This article explores the hidden forces at play—welding stress and deformation. Learn how these stresses impact strength, stability, and accuracy, and discover practical methods to minimize their effects. Get ready to understand the unseen challenges welders face and how to overcome them!
Welding stress refers to the internal forces generated within welded components during and after the welding process. These stresses are primarily caused by the localized heating and cooling cycles inherent to welding, which lead to non-uniform thermal expansion and contraction, as well as microstructural changes in the material.
The fundamental cause of welding stress and associated deformation lies in the heterogeneous temperature distribution during welding. This thermal gradient results in:
Welding stresses can be categorized into two main types:
In the absence of external loads, welding stresses are self-equilibrating within the weldment. However, these internal stresses can significantly impact the performance and quality of the welded structure in several ways:
① Effect on strength:
Serious defects in areas with high residual tensile stress can negatively impact the static load strength of the weldment if it operates below the brittle transition temperature. The presence of residual tensile stress at stress concentration points under cyclic stress will reduce the fatigue strength of the weldment.
The fatigue strength of weldments is not only dependent on the magnitude of residual stress, but also on factors such as the stress concentration factor, stress cycle characteristic coefficient, and the maximum value of cyclic stress. The influence of residual stress decreases as the stress concentration factor decreases and intensifies as the stress cycle characteristic coefficient decreases, but decreases as the cyclic stress increases.
When the cyclic stress approaches the yield strength, the effect of residual stress gradually diminishes.
② Effect on stiffness:
The combination of welding residual stress and stress from external loads can result in early yielding and plastic deformation in specific areas of the weldment. This will result in a reduction in the stiffness of the weldment.
③ Influence on the stability of pressure weldment:
When a welded rod is subjected to pressure, the welding residual stress combines with the stress from external loads, potentially causing local yielding or instability, and reducing the overall stability of the rod.
The impact of residual stress on stability depends on the geometry and distribution of internal stress within the member. The influence of residual stress on non-closed sections, such as I-sections, is greater than its influence on closed sections, such as box sections.
④ Influence on machining accuracy:
The presence of welding residual stress can affect the machining accuracy of weldments to varying degrees. The lower the stiffness of the weldment, the larger the machining amount, and the greater its impact on accuracy.
⑤ Influence on dimensional stability:
Welding residual stress and the size of the weldment both change over time, and this can affect the dimensional stability of the weldment. The stability of residual stress also influences the dimensional stability of the weldment.
⑥ Effect on corrosion resistance:
The combination of welding residual stress and load stress can result in stress corrosion cracking.
Influence of welding residual stress on structure and member:
Welding residual stress is the initial stress on a member before it bears any loads. During the use of the member, the residual stress overlaps with the working stress caused by other loads, leading to secondary deformation and a re-distribution of residual stress.
This not only decreases the stiffness and stability of the structure, but also significantly impacts its fatigue strength, resistance to brittle fracture, stress corrosion cracking, and high temperature creep cracking under the combined effects of temperature and the environment.
Welding stress can be minimized through comprehensive consideration of both design and process. In designing a welding structure, one should adopt welding joints with lower rigidity, minimize the quantity and cross-sectional size of welds, and avoid excessive concentration of welds. The following measures can be taken in terms of the process:
The determination of the welding sequence should allow the weld to shrink freely as much as possible to reduce stress. The welding stress generated by the sequence shown in Figure 4-10a is small, while in Figure 4-10b, welding seam 1 first increases the constraint on welding seam 2, thereby increasing the residual stress.
a) Minimal Welding Stress, b) Substantial Welding Stress.
During the cooling process of the weld, use a round-headed hammer to strike the weld evenly and quickly, causing local plastic elongation deformation of the weld metal, offsetting some of the welding shrinkage deformation, thereby reducing the residual welding stress.
Before welding, heat an appropriate part of the workpiece (known as the stress reduction zone) to elongate it (Figure 4-11). After welding, during cooling, the stress reduction zone and the weld shrink in the same direction, reducing welding stress and deformation.
The purpose of preheating before welding is to reduce the temperature difference between the welding zone and the surrounding metal, lower the cooling rate of the weld zone, and reduce the uneven expansion and contraction during welding heating and cooling, thereby reducing welding stress. Slow cooling after welding can have the same effect.
However, this method complicates the process and is only suitable for materials with poor plasticity and prone to cracking, such as high and medium carbon steel, cast iron, and alloy steel.
In order to eliminate the residual welding stress in the welding structure, stress relief annealing is commonly used in production. For carbon steel and low-to-medium alloy steel structures, the entire component or part of the welding joint can be heated to 600-800°C after welding, and slowly cooled after being kept at this temperature for a certain period of time. Generally, more than 80% of the residual welding stress can be eliminated.
To control welding deformation, the size and shape of the weld seams should be reasonably selected during the design of the welding structure, the number of weld seams should be minimized as much as possible, and the arrangement of weld seams should be symmetrical. In the production of welded structures, the following techniques can typically be applied:
Based on theoretical calculations and empirical values, the shrinkage allowance is considered in advance during the preparation and processing of the welding parts, so that the workpiece can achieve the required shape and size after welding.
Based on experience or measurement, the size and direction of structural welding deformation are estimated in advance. During the assembly of the welding structure, an intentional deformation is created in the opposite direction but equal in magnitude to offset the deformation produced after welding (see Figure 4-12).
a) Creation of angular deformation
b) Neutralization of angular deformation
The welding parts are fixed during welding and the rigid fixation is removed after the welding parts have cooled to room temperature. This can effectively prevent angular deformation and wave-like deformation, but it increases welding stress.
This method is only suitable for low-carbon steel structures with good plasticity and should not be used for cast iron and steel materials with a high tendency to harden to avoid post-welding fractures. Figure 4-13 shows the use of the rigid fixation method to prevent angular deformation of the flange face.
The selection of a reasonable welding sequence is essential for controlling welding deformation. For the welding of symmetrical section beams, the welding sequence shown in Figure 4-14 can effectively reduce welding deformation.
For workpieces with an uneven distribution of welds, like the main beam of the bridge crane shown in Figure 4-15, a sensible welding sequence is to have two workers simultaneously and symmetrically weld the 1-1′ seams first, followed by the 2-2′ seams, and finally the 3-3′ seams. In this way, the upward deformation caused by the 1-1′ weld can be essentially offset by the downward deflection caused by the 2-2 and 3-3 welds.
During the welding process, even when the measures mentioned above are taken, deformation exceeding the permissible value can sometimes occur. The commonly adopted methods for correcting welding deformation include:
Mechanical correction involves using external force to induce plastic deformation in the component in the opposite direction of the welding deformation, thus cancelling out each other’s deformation (Figure 4-16). This method is typically only suitable for low-carbon steel and ordinary low-alloy steel that have relatively low rigidity and good plasticity.
Flame correction uses the cooling shrinkage after local heating of the metal to correct the existing welding deformation. Figure 4-17 shows an upward deformation of a T-beam after welding, which can be corrected by heating the web position with a flame. The heating zone is triangular, and the heating temperature is 600-800°C.
After cooling, the web shrinks, causing reverse deformation and straightening the welded component. This method is mainly suitable for materials with good plasticity and no hardening tendency.
Vibration stress relief (VSR) is an advanced technique for reducing residual stresses in welded structures and engineering materials. This process involves applying controlled, low-frequency vibrations to the workpiece, typically in the range of 20-100 Hz. When the combined effect of residual stress and induced vibrational stress exceeds the material’s yield strength locally, localized plastic deformation occurs, leading to stress redistribution and overall reduction.
The effectiveness of VSR depends on several factors:
VSR offers several advantages over thermal stress relief methods:
However, it’s important to note that VSR may not be suitable for all materials and geometries, and its effectiveness can vary depending on the specific application.
Thermal stress relief, also known as post-weld heat treatment (PWHT), is a well-established method for reducing residual stresses in welded components. The process involves carefully controlled heating, soaking, and cooling cycles:
Key considerations for effective thermal stress relief:
While thermal stress relief is highly effective, improper execution can lead to detrimental effects:
To ensure optimal results, it’s crucial to:
By carefully controlling these parameters and following established best practices, thermal stress relief can effectively reduce residual stresses, improving the dimensional stability and fatigue resistance of welded structures.