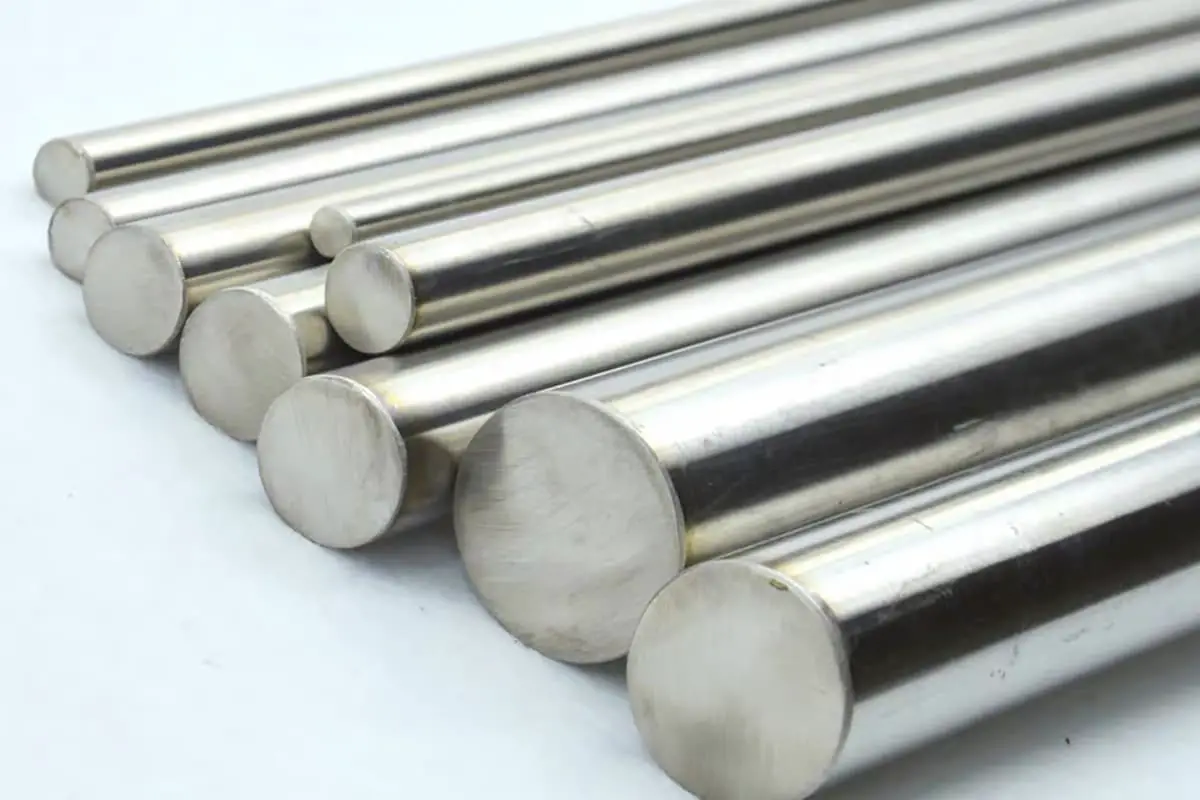
Imagine a world without steel plates. From the car you drive to the bridges you cross, these unassuming slabs form the backbone of modern infrastructure. In this article, we unravel the diverse types of steel plates, their varied thicknesses, and crucial applications. Discover how different processes and compositions give rise to steel plates tailored for everything from shipbuilding to pressure vessels. Ready to explore the material that shapes our world? Read on to learn how steel plates are classified and utilized across industries.
Steel plate is a flat steel material that is poured with molten steel and pressed after cooling.
Steel plates are flat, rectangular, and can be directly rolled or cut from wide steel strips.
Steel plates are classified by thickness: thin plates are less than 4 millimeters (with a minimum thickness of 0.2 millimeters), medium-thick plates range from 4 to 60 millimeters, and heavy plates range from 60 to 115 millimeters.
Steel plates are categorized by rolling process: hot-rolled and cold-rolled.
The width of thin plates ranges from 500 to 1500 millimeters, while the width of thick plates ranges from 600 to 3000 millimeters.
Thin plates are classified based on the type of steel, including common steel, high-quality steel, alloy steel, spring steel, stainless steel, tool steel, heat-resistant steel, bearing steel, silicon steel, and industrial pure iron plates.
They can also be classified based on specific applications, such as oil drum plates, enamel plates, bulletproof plates, etc.
Furthermore, they can be categorized based on the surface coating, including galvanized plates, tin-plated plates, lead-plated plates, plastic composite steel plates, and more.
The steel grades for thick steel plates are generally similar to those for thin steel plates.
However, in terms of specific applications, there are some varieties of steel plates that are primarily used for thick plates, such as bridge steel plates, boiler steel plates, automobile manufacturing steel plates, pressure vessel steel plates, and multi-layer high-pressure vessel steel plates.
However, there are some varieties of steel plates that overlap with thin plates, such as automobile beam steel plates (2.5-10 millimeters thick), patterned steel plates (2.5-8 millimeters thick), stainless steel plates, heat-resistant steel plates, etc.
Furthermore, steel plates can have different material compositions. Not all steel plates are the same, and their usage depends on the specific material composition.
With the development of science, technology, and industry, higher requirements have been placed on materials, such as higher strength, resistance to high temperature, high pressure, low temperature, corrosion resistance, wear resistance, and other special physical and chemical properties. Carbon steel alone cannot fully meet these requirements.
(1) Low hardenability: In general, the maximum hardenability of carbon steel water quenching is only about 10mm-20mm in diameter.
(2) Low strength and yield ratio: For example, the yield strength (σs) of ordinary carbon steel Q235 is 235 MPa, while low-alloy structural steel 16Mn has a yield strength (σs) of over 360 MPa. The σs/σb ratio of 40 steel is only 0.43, much lower than that of alloy steel.
(3) Poor tempering stability: Due to poor tempering stability, when carbon steel undergoes tempering treatment, lower tempering temperatures are required to achieve higher strength, resulting in lower toughness. On the other hand, higher tempering temperatures are required to achieve better toughness, but this results in lower strength. Therefore, the overall mechanical properties of carbon steel are not high.
(4) Inability to meet special performance requirements: Carbon steel often lacks good properties in terms of oxidation resistance, corrosion resistance, heat resistance, low-temperature resistance, wear resistance, and special electromagnetic properties. It cannot meet the demands for special performance requirements.
Based on the content of alloying elements, alloy steel can be classified into:
Based on the main alloying elements, alloy steel can be further classified into:
Based on the microstructure of small test samples after normalizing or as-cast, alloy steel can be classified into:
Based on the application, alloy steel can be categorized as:
The carbon content is indicated by a numerical value at the beginning of the grade. For structural steel, the carbon content is expressed in two decimal places as a unit of ten thousandth (e.g., 45 represents a carbon content of 0.0045%).
For tool steel and special performance steel, the carbon content is expressed in one decimal place as a unit of one thousandth, except when the carbon content exceeds 1%.
After indicating the carbon content, the chemical symbols of the main alloying elements are used to represent their content, followed by numerical values. If the average content is less than 1.5%, it is not indicated. If the average content is between 1.5% and 2.49%, 2.5% and 3.49%, and so on, it is indicated as 2, 3, and so forth.
For example, 40Cr represents an average carbon content of 0.40% and a chromium content below 1.5%. 5CrMnMo represents an average carbon content of 0.5% and the contents of chromium, manganese, and molybdenum below 1.5%.
For special-purpose steel, the Chinese pinyin prefix indicating its application is used. For example, “G” is added before the steel grade to indicate bearing steel. GCr15 represents bearing steel with a carbon content of about 1.0% and a chromium content of about 1.5% (which is an exception, as the chromium content is expressed in thousandths).
Y40Mn represents free-cutting steel with a carbon content of 0.4% and a manganese content below 1.5%.
For high-quality steel, the letter “A” is added at the end of the steel grade. For example, 20Cr2Ni4A.
After adding alloying elements to steel, there is an interaction between the base elements of iron and carbon and the added alloying elements.
The purpose of alloying steel is to utilize the interaction between alloying elements and iron-carbon, as well as their effects on the iron-carbon phase diagram and heat treatment, to improve the structure and properties of the steel.
After alloying elements are added to steel, they mainly exist in three forms: dissolved in iron, forming carbides, or in high-alloy steels, possibly forming intermetallic compounds.
1. Dissolved in iron:
Almost all alloying elements (except Pb) can dissolve into iron, forming alloy ferrite or alloy austenite. According to their effects on α-Fe or γ-Fe, alloying elements can be classified into two categories: elements that expand the γ-phase region and elements that contract the γ-phase region.
Elements that expand the γ-phase region, also known as austenite stabilizers, include Mn, Ni, Co, C, N, Cu, etc. They lower the A3 temperature (transformation temperature of γ-Fe to α-Fe) and raise the A4 temperature (transformation temperature of γ-Fe). This expands the range of the γ-phase.
Among them, Ni and Mn, when added in certain amounts, can expand the γ-phase region below room temperature, causing the α-phase region to disappear. They are referred to as elements that completely expand the γ-phase region.
Other elements such as C, N, Cu, etc., can partially expand the γ-phase region but not to room temperature, so they are called elements that partially expand the γ-phase region.
Elements that contract the γ-phase region, also known as ferrite stabilizers, include Cr, Mo, W, V, Ti, Al, Si, B, Nb, Zr, etc. They raise the A3 temperature and lower the A4 temperature (except for chromium, where A3 temperature decreases when chromium content is below 7%, but increases rapidly when it exceeds 7%).
This contracts the range of the γ-phase, enlarging the region of stability for ferrite. Based on their effects, they can be further classified into elements that completely contract the γ-phase region (e.g., Cr, Mo, W, V, Ti, Al, Si) and elements that partially contract the γ-phase region (e.g., B, Nb, Zr).
2. Alloying elements that form carbides can be categorized into two major groups, based on their affinity for carbon in steel: carbide-forming elements and non-carbide-forming elements.
Common non-carbide-forming elements include Ni, Co, Cu, Si, Al, N, and B. These essentially dissolve in ferrite and austenite. Common carbide-forming elements include Mn, Cr, W, V, Nb, Zr, Ti, etc. (arranged in order of the stability of the carbides formed, from weakest to strongest). In steel, part of them dissolve in the matrix phase, and part form alloy carbides. When present in high amounts, they can form new alloy carbides.
Effects on the range of austenite and ferrite:
Elements that expand or shrink the γ phase region also have a similar effect on the γ phase region in the Fe-Fe3C phase diagram. When the content of Ni or Mn is high, it can result in single-phase austenite structure at room temperature (e.g., 1Cr18Ni9 austenitic stainless steel and ZGMn13 high manganese steel).
On the other hand, when Cr, Ti, Si, etc., exceed a certain concentration, it can lead to the formation of single-phase ferrite structure at room temperature (e.g., 1Cr17Ti high-chromium ferritic stainless steel).
Effects on the critical points (S and E points) in the Fe-Fe3C phase diagram:
Elements that expand the γ phase region lower the eutectoid transformation temperature (S point) in the Fe-Fe3C phase diagram, while elements that shrink the γ phase region raise the eutectoid transformation temperature.
Almost all alloying elements reduce the carbon content at the eutectoid point (S) and eutectic point (E), shifting them to the left. The effect of strong carbide-forming elements is particularly significant.
Effects on steel heat treatment:
Alloying elements can influence the phase transformations that occur during heat treatment of steel.
1. Effects on phase transformation during heating:
Alloying elements can affect the rate of austenite formation and the size of austenite grains during heating.
(1) Influence on the rate of austenite formation:
Strong carbide-forming elements like Cr, Mo, W, V form insoluble alloy carbides in austenite, significantly slowing down the formation of austenite. Partial non-carbide-forming elements like Co, Ni enhance carbon diffusion, accelerating the formation of austenite. Alloying elements like Al, Si, Mn have little effect on the rate of austenite formation.
(2) Influence on the size of austenite grains:
Most alloying elements hinder the growth of austenite grains, but to varying degrees. Elements like V, Ti, Nb, Zr strongly hinder grain growth, while elements like W, Mn, Cr moderately hinder grain growth. Elements like Si, Ni, Cu have little effect on grain growth, while elements like Mn, P promote grain growth.
2. Effects on the transformation of supercooled austenite:
Except for Co, almost all alloying elements increase the stability of supercooled austenite, delaying the transformation to pearlite. This results in a rightward shift of the C-curve, indicating improved hardenability of the steel.
Common elements used to enhance hardenability include Mo, Mn, Cr, Ni, Si, B. It should be noted that the alloying elements can only enhance hardenability if they are completely dissolved in austenite. If not fully dissolved, carbides can become the nucleus of pearlite, reducing hardenability.
Additionally, the combined addition of multiple alloying elements (e.g., Cr-Mn steel, Cr-Ni steel) has a much stronger effect on hardenability than individual elements.
Except for Co and Al, most alloying elements lower the Ms and Mf points. The order of their influence is Mn, Cr, Ni, Mo, W, Si. Among them, Mn has the strongest effect, while Si has no practical effect.
The lowering of Ms and Mf points increases the amount of retained austenite after quenching. When there is an excessive amount of retained austenite, it can undergo sub-zero treatment (cooling below the Mf point) to transform it into martensite or undergo multiple tempering processes.
In the latter case, the precipitation of alloy carbides from the retained austenite can cause the Ms and Mf points to rise, and during cooling, it can transform into martensite or bainite (a process known as secondary hardening).
3. Effects on tempering transformation:
(1) Improved tempering stability:
Alloying elements can delay the decomposition of martensite and the transformation of retained austenite during tempering (i.e., they start decomposing and transforming at higher temperatures). They also raise the recrystallization temperature of ferrite, making it difficult for carbides to coalesce and grow.
As a result, the resistance of the steel to tempering softening is increased, enhancing its tempering stability. Alloying elements with a strong effect on tempering stability include V, Si, Mo, W, Ni, Co.
(2) Secondary hardening:
In some high-alloy steels with high contents of Mo, W, V, the hardness does not monotonically decrease with increasing tempering temperature but instead starts to increase after reaching a certain temperature (around 400°C) and reaches a peak at a higher temperature (generally around 550°C).
This phenomenon is known as secondary hardening during tempering and is related to the nature of the precipitates formed during tempering. When the tempering temperature is below 450°C, carbides precipitate in the steel.
Above 450°C, the carbides dissolve, and dispersed stable refractory carbides such as Mo2C, W2C, VC precipitate, causing the hardness to increase again. This is known as precipitation hardening.
Secondary hardening can also occur due to the secondary quenching of retained austenite during the cooling process after tempering.
Elements causing secondary hardening:
Causes of secondary hardening: Alloying elements
Transformation of retained austenite: Precipitation hardening: Mn, Mo, W, Cr, Ni, Co①, V V, Mo, W, Cr, Ni, Co.
①Effective only at high concentrations and in the presence of other alloying elements capable of forming dispersed intermetallic compounds.
(3) Increased temper brittleness:
Like carbon steel, alloy steel can exhibit temper brittleness, and it is often more pronounced. This is an adverse effect of alloying elements. The second type of temper brittleness (high-temperature temper brittleness) that occurs between 450°C and 600°C is primarily associated with severe segregation of certain impurity elements and the alloying elements themselves at the original austenite grain boundaries.
It commonly occurs in alloy steels containing elements such as Mn, Cr, Ni. This type of brittleness is reversible, and rapid cooling (usually oil quenching) after tempering can prevent its occurrence. The addition of appropriate amounts of Mo or W (0.5% Mo, 1% W) can also effectively eliminate this type of brittleness.
Effects of alloying elements on the mechanical properties of steel:
One of the main purposes of adding alloying elements is to increase the strength of the steel. To enhance strength, efforts are made to increase the resistance to dislocation movement.
The main strengthening mechanisms in metals are solid solution strengthening, dislocation strengthening, grain refinement strengthening, and second-phase (precipitate and dispersion) strengthening. Alloying elements leverage these strengthening mechanisms to achieve their strengthening effects.
1. Effects on the mechanical properties of steel in the annealed state:
In the annealed state, the basic phases in structural steel are ferrite and carbides. Alloying elements dissolve in ferrite, forming alloyed ferrite and achieving strength and hardness enhancement through solid solution strengthening. However, this also reduces the plasticity and toughness of the steel.
2. Effects on the mechanical properties of steel in the normalized state:
The addition of alloying elements lowers the carbon content at the eutectoid point, shifting the C-curve to the right. This increases the proportion of pearlite in the microstructure and reduces the interlamellar spacing, resulting in increased strength and decreased ductility. However, in the normalized state, alloy steel does not exhibit significant superiority compared to carbon steel.
3. Effects on the mechanical properties of steel in the quenched and tempered state:
The strengthening effect of alloying elements is most significant in quenched and tempered steel because it fully utilizes all four strengthening mechanisms. During quenching, martensite is formed, and during tempering, carbides precipitate, resulting in strong second-phase strengthening.
Simultaneously, it greatly improves the toughness of the steel. Therefore, obtaining martensite and tempering it is the most economical and effective method for comprehensive strengthening of steel.
The primary purpose of adding alloying elements to steel is to enhance its hardenability, ensuring easy formation of martensite during quenching.
The secondary purpose is to improve tempering stability, allowing the retention of martensite to higher temperatures and enabling the formation of finer, more uniform, and stable precipitated carbides during tempering. As a result, alloy steel exhibits higher strength than carbon steel under similar conditions.
Impact of Alloy Elements on the Technological Properties of Steel
1. Impact of Alloy Elements on the Castability of Steel
The lower the temperature of the solid and liquid phase lines and the narrower the crystallization temperature range, the better the castability of the steel. The impact of alloy elements on castability primarily depends on their effect on the Fe-Fe3C phase diagram.
Moreover, many elements such as Cr, Mo, V, Ti, Al form high melting point carbides or oxide particles in steel, which increase the viscosity of the steel, reduce its fluidity, and deteriorate its castability.
2. Impact of Alloy Elements on the Plastic Formability of Steel
Plastic forming includes hot and cold processing. Alloy elements dissolved in solid solution, or forming carbides (such as Cr, Mo, W, etc.), increase the resistance to thermal deformation of the steel and significantly decrease its hot plasticity, making it prone to forging cracks. The hot forming processability of general alloy steel is much worse than that of carbon steel.
3. Impact of Alloy Elements on the Weldability of Steel
Alloy elements increase the hardenability of steel, promote the formation of brittle structures (martensite), and deteriorate the weldability. However, the presence of a small amount of Ti and V in the steel can improve its weldability.
4. Impact of Alloy Elements on the Machinability of Steel
Machinability is closely related to the hardness of the steel, and the suitable hardness range for cutting processing of steel is 170HB to 230HB. In general, the machinability of alloy steel is worse than that of carbon steel. However, the addition of S, P, Pb and other elements can significantly improve the machinability of steel.
5. Impact of Alloy Elements on the Heat Treatment Processability of Steel
Heat treatment processability reflects the difficulty of steel heat treatment and the tendency to produce defects. It mainly includes hardenability, overheating sensitivity, temper brittleness tendency, and oxidation decarburization tendency.
Alloy steel has high hardenability, and a relatively slow cooling method can be used during quenching to reduce the deformation and cracking tendency of the workpiece. The addition of manganese and silicon increases the overheating sensitivity of the steel.
The type of steel used for manufacturing important engineering structures and machine parts is known as alloy structural steel. This mainly includes low alloy structural steel, alloy carburizing steel, alloy quenching and tempering steel, alloy spring steel, and rolling bearing steel.
(Also known as Common Low-Alloy Steel, HSLA)
1. Applications
Primarily used in the manufacturing of bridges, ships, vehicles, boilers, high-pressure vessels, oil and gas transmission pipelines, large steel structures, and more.
2. Performance Requirements
(1) High Strength: The yield strength is generally above 300MPa.
(2) High Toughness: An elongation rate of 15%-20% is required, with room temperature impact toughness exceeding 600kJ/m to 800kJ/m. For large welded components, higher fracture toughness is necessary.
(3) Good weldability and cold forming capabilities.
(4) Low cold brittle transition temperature.
(5) Excellent corrosion resistance.
3. Composition Characteristics
(1) Low Carbon: Due to the high requirements for toughness, weldability, and cold formability, the carbon content should not exceed 0.20%.
(2) The addition of manganese as the primary alloying element.
(3) Addition of niobium, titanium, or vanadium as auxiliary elements: Small quantities of niobium, titanium, or vanadium forming fine carbides or carbonitrides in the steel, contribute to fine ferrite grains and improve the steel’s strength and toughness. Furthermore, adding small amounts of copper (≤0.4%) and phosphorus (around 0.1%) enhances corrosion resistance. The inclusion of trace rare earth elements facilitates desulphurization and degassing, purifying the steel and improving its toughness and workability.
4. Common Low-Alloy Structural Steels
16Mn is the most widely used and produced type of low-alloy high-strength steel in China. It is a ferrite-pearlite structure with fine grains, offering a strength approximately 20%-30% higher than common carbon structural steel Q235, and a 20%-38% higher atmospheric corrosion resistance.
15MnVN is the most commonly used type of medium-grade strength steel. It exhibits higher strength, along with good toughness, weldability, and low-temperature toughness, making it extensively used in the manufacturing of bridges, boilers, ships, and other large structures.
Once the strength level exceeds 500MPa, ferrite and pearlite structures are insufficient, thus, low carbon bainite steel is developed. The addition of elements like Cr, Mo, Mn, B helps to form a bainitic structure under air cooling conditions, providing higher strength, better plasticity, and weldability, often utilized in high-pressure boilers, high-pressure vessels, etc.
5. Heat Treatment Characteristics
This type of steel is generally used in a hot-rolled air-cooled state, without the need for specific heat treatment. The microstructure in the working state is typically ferrite + sorbite.
1. Applications
Primarily used in manufacturing machine parts such as transmission gears in automobiles and tractors, camshafts and piston pins in internal combustion engines. These parts endure intense friction and wear in operation, and simultaneously bear considerable alternating loads, particularly impact loads.
2. Performance Requirements
(1) The carburized surface layer has high hardness to ensure excellent wear resistance and contact fatigue resistance, while maintaining appropriate plasticity and toughness.
(2) The core has high toughness and sufficiently high strength. If the core’s toughness is inadequate, it can easily fracture under impact loads or overload; if the strength is insufficient, the brittle carburized layer can fracture and peel off.
(3) Good heat treatment processability. At high carburizing temperatures (900℃ to 950℃), austenite grains do not easily grow, and have good hardenability.
3. Composition Characteristics
(1) Low Carbon: The carbon content is typically 0.10% to 0.25%, which ensures sufficient plasticity and toughness in the part core.
(2) Addition of hardenability improving alloying elements: Common additions include Cr, Ni, Mn, B, etc.
(3) Addition of elements to inhibit the growth of austenite grains: This typically involves adding small amounts of strong carbide-forming elements such as Ti, V, W, Mo, etc., to form stable alloy carbides.
4. Types and Grades of Steel
20Cr is a low hardenability alloy carburized steel. The hardenability of this type of steel is low, with lower core strength.
20CrMnTi is a medium hardenability alloy carburized steel. This type of steel has higher hardenability, less overheating sensitivity, a uniform carburization transition layer, and exhibits good mechanical and processing properties.
18Cr2Ni4WA and 20Cr2Ni4A are high hardenability alloy carburized steels. These types of steel contain more Cr, Ni elements, have very high hardenability, and demonstrate excellent toughness and low-temperature impact toughness.
5. Heat Treatment and Organizational Performance
The heat treatment process for alloy carburized steel typically involves carburizing followed by direct quenching, and then low-temperature tempering. After heat treatment, the surface carburized layer consists of alloy carbides + tempered martensite + a small amount of residual austenite, with a hardness of 60HRC to 62HRC.
The core structure is related to the steel’s hardenability and the part’s cross-sectional dimensions. When fully hardened, it is low carbon tempered martensite, with a hardness of 40HRC to 48HRC; in most cases, it is bainite, tempered martensite, and a small amount of ferrite, with a hardness of 25HRC to 40HRC. The core’s toughness is generally higher than 700KJ/m2.
1. Applications
Alloy quenched and tempered steel is widely used to manufacture various critical parts in automobiles, tractors, machine tools, and other machines, such as gears, shafts, connecting rods, and bolts.
2. Performance Requirements
Most of the tempered parts bear multiple workloads, the stress situation is relatively complex, requiring high comprehensive mechanical properties, i.e., high strength, good plasticity, and toughness. Alloy quenched and tempered steel also needs to have good hardenability. However, different parts have different stress conditions, so the requirements for hardenability are different.
3. Composition Characteristics
(1) Medium Carbon: The carbon content is generally between 0.25% and 0.50%, with 0.4% being the most common.
(2) Addition of elements Cr, Mn, Ni, Si, etc., to improve hardenability: These alloy elements not only improve hardenability but also form alloy ferrite, enhancing the strength of steel. For instance, the performance of 40Cr steel after quenching and tempering is much higher than that of 45 steel.
(3) Addition of elements to prevent second-class tempering brittleness: Alloy quenched and tempered steel containing Ni, Cr, Mn, is prone to second-class tempering brittleness when slow cooled after high-temperature tempering. Adding Mo, W to steel can prevent second-class tempering brittleness, with an appropriate content of about 0.15% to 0.30% Mo or 0.8% to 1.2% W.
Comparison of the performance of 45 steel and 40Cr steel after quenching and tempering:
4. Types and Grades of Steel
(1) 40Cr low hardenability quenched and tempered steel: The oil quenching critical diameter of this type of steel is 30mm to 40mm, used for manufacturing general-sized critical parts.
(2) 35CrMo medium hardenability alloy quenched and tempered steel: The oil quenching critical diameter of this type of steel is 40mm to 60mm, adding molybdenum not only improves hardenability but also prevents second-class tempering brittleness.
(3) 40CrNiMo high hardenability alloy quenched and tempered steel: The oil quenching critical diameter of this type of steel is 60mm to 100mm, mostly CrNi steel. Adding an appropriate amount of molybdenum to CrNi steel not only provides good hardenability but also eliminates second-class tempering brittleness.
5. Heat Treatment and Organizational Performance
The final heat treatment of alloy quenched and tempered steel is quenching plus high-temperature tempering (quenching and tempering treatment). Alloy quenched and tempered steel has higher hardenability, usually oil quenched, and when hardenability is particularly large, even air cooling can be used, reducing heat treatment defects.
The final performance of alloy quenched and tempered steel depends on the tempering temperature. Generally, tempering at 500℃-650℃ is adopted. By selecting the tempering temperature, the required performance can be obtained. To prevent second-class tempering brittleness, rapid cooling after tempering (water cooling or oil cooling) is beneficial to improve toughness.
The structure of alloy quenched and tempered steel after conventional heat treatment is tempered sorbite. For parts requiring wear resistance on the surface (such as gears and spindles), induction heating surface quenching and low-temperature tempering are carried out, and the surface structure is tempered martensite. The surface hardness can reach 55HRC to 58HRC.
The yield strength of alloy quenched and tempered steel after quenching and tempering is about 800MPa, the impact toughness is about 800kJ/m2, and the core hardness can reach 22HRC to 25HRC. If the cross-sectional size is large and not quenched, the performance is significantly reduced.
By thickness:
(1) Thin plate, thickness not exceeding 3mm (excluding electrical steel plate)
(2) Medium plate, thickness between 4-20mm
(3) Thick plate, thickness between 20-60mm
(4) Extra-thick plate, thickness greater than 60mm
By production method:
(1) Hot-rolled steel plate
(2) Cold-rolled steel plate
By surface characteristics:
(1) Galvanized plate (Hot-dip galvanized plate, Electro-galvanized plate)
(2) Tin-plated plate
(3) Composite steel plate
(4) Color-coated steel plate
By use:
(1) Bridge steel plate
(2) Boiler steel plate
(3) Shipbuilding steel plate
(4) Armor steel plate
(5) Automobile steel plate
(6) Roofing steel plate
(7) Structural steel plate
(8) Electrical steel plate (Silicon steel sheet)
(9) Spring steel plate
(10) Heat-resistant steel plate
(11) Alloy steel plate
(12) Other
Common brands in general and mechanical structural steel plates
1. In Japanese steel materials (JIS series), the brand name of common structural steel consists of three parts:
For example, SS400 – the first S stands for steel, the second S represents “structure”, and 400 is the minimum tensile strength of 400MPa, collectively representing common structural steel with a tensile strength of 400 MPa.
2. SPHC – the initial S stands for Steel, P stands for Plate, H stands for Heat, C stands for Commercial, collectively indicating general use hot-rolled steel plate and strip.
3. SPHD – represents stamping use hot-rolled steel plate and strip.
4. SPHE – represents deep drawing use hot-rolled steel plate and strip.
5. SPCC – represents general use cold-rolled carbon steel thin plate and strip. The third letter C is the abbreviation for Cold. For ensuring the tensile test, T is added at the end of the brand to become SPCCT.
6. SPCD – represents stamping use cold-rolled carbon steel thin plate and strip, equivalent to China’s 08AL (13237) high-quality carbon structural steel.
7. SPCE – represents deep drawing use cold-rolled carbon steel thin plate and strip, equivalent to China’s 08AL (5213) deep drawing steel. To ensure non-aging, N is added at the end of the brand to become SPCEN.
Cold-rolled carbon steel thin plate and strip temper designation: Annealed state is A, standard temper is S, 1/8 hard is 8, 1/4 hard is 4, 1/2 hard is 2, hard is 1.
Surface finishing designation: Dull finish rolling is D, bright finish rolling is B. For example, SPCC-SD represents standard temper, dull finish rolling general use cold-rolled carbon thin plate. Another example, SPCCT-SB represents standard temper, bright finishing, requiring guaranteed mechanical properties of cold-rolled carbon thin plate.
8. The representation method of JIS mechanical structural steel brand name is: S + carbon content + letter code (C, CK), where the carbon content is represented by the median value x 100, letter C stands for carbon, and K stands for carburizing steel. For instance, coiled carbon steel S20C has a carbon content of 0.18-0.23%.
Silicon Steel Brand
1. China brand representation method:
(1) Cold-rolled non-oriented silicon steel strip (sheet): Representation method: DW + iron loss value (iron loss value per unit weight at a peak magnetic flux of 1.5T with a frequency of 50HZ and a sine waveform.) multiplied by 100 + thickness value multiplied by 100. For instance, DW470-50 represents cold-rolled non-oriented silicon steel with an iron loss value of 4.7w/kg and thickness of 0.5mm, and the new model is represented as 50W470.
(2) Cold-rolled oriented silicon steel strip (sheet): Representation method: DQ + iron loss value (iron loss value per unit weight at a peak magnetic flux of 1.7T with a frequency of 50HZ and a sine waveform.) multiplied by 100 + thickness value multiplied by 100. Sometimes a G is added after the iron loss value to represent high magnetic induction.
(3) Hot-rolled silicon steel plate: Hot-rolled silicon steel plate is represented by DR, and according to the silicon content, it is divided into low silicon steel (silicon content ≤2.8%) and high silicon steel (silicon content >2.8%).
Representation method: DR + iron loss value (iron loss value per unit weight at a peak magnetic flux of 1.5T with a frequency of 50HZ and a sine waveform.) multiplied by 100 + thickness value multiplied by 100. For instance, DR510-50 represents hot-rolled silicon steel plate with an iron loss value of 5.1 and thickness of 0.5mm.
The brand name of hot-rolled silicon thin plate for household appliances is represented by JDR + iron loss value + thickness value, such as JDR540-50.
2. Japan brand representation method:
(1) Cold-rolled non-oriented silicon steel strip: Consists of nominal thickness (value multiplied by 100) + code A + iron loss guarantee value (value of iron loss at a maximum magnetic flux density of 1.5T with a frequency of 50HZ, multiplied by 100). For instance, 50A470 represents a cold-rolled non-oriented silicon steel strip with a thickness of 0.5mm and an iron loss guarantee value of ≤4.7.
(2) Cold-rolled oriented silicon steel strip: Composed of nominal thickness (value multiplied by 100) + code G: represents ordinary material, P: represents highly oriented material + iron loss guarantee value (value of iron loss at a maximum magnetic flux density of 1.7T with a frequency of 50HZ, multiplied by 100). For example, 30G130 represents a cold-rolled oriented silicon steel strip with a thickness of 0.3mm and an iron loss guarantee value of ≤1.3.
Tin-plated Plate and Hot-dip Galvanized Plate
1. Tin-plated plate: Electrolytic tinplate and strip, also known as tinned iron, this steel plate (strip) is coated with tin on the surface, has good corrosion resistance, and is non-toxic, can be used as packaging material for cans, cable sheathing, instrument and telecommunication parts, battery and other small hardware.
The classification and symbols of tin-plated steel plates and strips are as follows:
Classification method | Category | Symbol |
By the amount of tin plating | Uniform Tin Plating E1, E2, E3, E4 | |
By hardness grade | T50, T52, T57, T61, T65, T70 | |
By surface condition | Smooth Surface | G |
Stone patterned surface | S | |
Linen patterned surface | M | |
By passivation method | Low Chromium Passivation | L |
Chemical passivation | H | |
Cathodic electrochemical passivation | Y | |
By oiling amount | Lightly oil. | Q |
Heavy oiling | Z | |
By surface quality | One set | I |
Group two | II |
The specifications for uniform and differential tin plating thickness are as follows:
symbol | Nominal tin plating amount, g/m2 | Minimum average tin plating amount g/m2 |
E1 | 5.6(2.8/2.8) | 4.9 |
E2 | 11.2(5.6/5.6) | 10.5 |
E3 | 16.8(8.4/8.4) | 15.7 |
E4 | 22.4(11.2/11.2) | 20.2 |
D1 | 5.6/2.8 | 5.05/2.25 |
D2 | 8.4/2.8 | 7.85/2.25 |
D3 | 8.4/5.6 | 7.85/5.05 |
D4 | 11.2/2.8 | 10.1/2.25 |
D5 | 11.2/5.6 | 10.1/5.05 |
D6 | 11.2/8.4 | 10.1/7.85 |
D7 | 15.1/5.6 | 13.4/5.05 |
2. Hot-dip galvanized sheet: A zinc coat is applied on the surface of thin steel plates and steel strips through a continuous hot-dip process, which can prevent the surface of the thin steel plates and steel strips from corroding and rusting.
Galvanized steel plates and steel strips are widely used in industries such as machinery, light industry, construction, transportation, chemical, and telecommunications. The classification and symbols of galvanized steel plates and steel strips are displayed in the table below:
Classification method | Category | Symbol | |
According to processing performance | General purpose | PT | |
Mechanical occlusion | JY | ||
Deep drawing | SC | ||
Ultra deep drawing and aging resistance | CS | ||
structure | JG | ||
By weight of zinc layer | zinc | 001 | 001 |
100 | 100 | ||
200 | 200 | ||
275 | 275 | ||
350 | 350 | ||
450 | 450 | ||
600 | 600 | ||
Zinc iron alloy | 001 | 001 | |
90 | 90 | ||
120 | 120 | ||
180 | 180 | ||
By surface structure: | Normal Zinc Flower | Z | |
Small zinc flower | X | ||
Smooth zinc flower | GZ | ||
Zinc-iron alloy | XT | ||
By surface quality: | IGROUP | I | |
Group II | II | ||
By dimensional accuracy: | Advanced Precision | A | |
General precision | B | ||
By surface treatment: | Chromic Acid Passivation | L | |
Oil coating | Y | ||
Chromic acid passivation plus oil coating | LY |
The zinc layer of No. 001 weighs less than 100g/m2.
Boiling Plate Steel vs Calm Plate Steel
1. Boiling plate steel is hot-rolled from ordinary carbon structural steel, also known as boiling steel.
This type of steel is partially deoxidized, using only a certain amount of weak deoxidizer, resulting in high oxygen content in the molten steel. Upon pouring the steel into the ingot mold, a reaction between carbon and oxygen produces copious gases, causing the steel to boil, thus the name. Boiling steel has a low carbon content and low silicon content due to the absence of deoxidation by ferrosilicon (Si<0.07%).
The outer layer of boiling steel is crystallized under the conditions of intense agitation caused by boiling, resulting in a pure, dense surface with good quality, excellent plasticity, and stamping performance. There are no significant concentrated shrinkage holes, fewer cutting heads, a high yield rate, and a low cost due to simple production processes and minimal consumption of ferroalloy. Boiling steel plates are used extensively in the manufacture of various stamping parts, building and engineering structures, and some less important machine structure components.
However, boiling steel has several impurities at its core, significant segregation, non-compact organization, and uneven mechanical properties. Due to its high gas content, its toughness is low, it has high cold brittleness, and sensitivity to aging, and its weldability is poor. Therefore, boiling steel plates are unsuitable for manufacturing structures that bear impact loads, work under low-temperature conditions, and other critical structures.
2. Calm plate steel is hot-rolled from ordinary carbon structural steel, known as calm steel.
This is a fully deoxidized steel where the molten steel is deoxidized thoroughly using ferromanganese, ferrosilicon, and aluminum before casting, resulting in low oxygen content (generally 0.002-0.003%). The molten steel remains calm in the ingot mold, without boiling, hence the name.
Under normal operating conditions, calm steel has no bubbles, and its structure is uniformly dense. Due to the low oxygen content, there are fewer oxide inclusions in the steel, the purity is higher, and cold brittleness and aging tendency are small. Also, calm steel has smaller segregation, more uniform properties, and higher quality. The disadvantage of calm steel is the presence of concentrated shrinkage holes, a low yield rate, and a high price. Therefore, calm steel materials are primarily used in components that can withstand impacts at low temperatures, welding structures, and other components that require high strength.
Low alloy steel plates are calm steel and semi-calm steel plates. Due to their high strength, superior performance, and considerable steel saving, which reduces structural weight, their application has become increasingly extensive.
Carbon Structural Steel Plates
High-quality carbon structural steel is carbon steel with a carbon content of less than 0.8%. This type of steel contains less sulfur, phosphorus, and non-metallic inclusions than carbon structural steel, resulting in superior mechanical performance.
High-quality carbon structural steel is divided into three categories based on carbon content: low carbon steel (C≤0.25%), medium carbon steel (C=0.25-0.6%), and high carbon steel (C>0.6%).
High-quality carbon structural steel is divided into two groups based on manganese content: regular manganese content (manganese 0.25%-0.8%) and high manganese content (manganese 0.70%-1.20%), with the latter having better mechanical properties and processability.
1. Hot Rolled Thin Steel Plate and Steel Strip of High-Quality Carbon Structural Steel:
These are used in automotive, aviation industries, and other sectors. The steel grades include boiling steel: 08F, 10F, 15F; calm steel: 08, 08AL, 10, 15, 20, 25, 30, 35, 40, 45, 50. Grades 25 and below are low carbon steel plates, and 30 and above are medium carbon steel plates.
2. Hot Rolled Thick Steel Plate and Wide Steel Strip of High-Quality Carbon Structural Steel:
These are used for various mechanical structure components. The steel grades include low carbon steel: 05F, 08F, 08, 10F, 10, 15F, 15, 20F, 20, 25, 20Mn, 25Mn, etc.; medium carbon steel: 30, 35, 40, 45, 50, 55, 60, 30Mn, 40Mn, 50Mn, 60Mn, etc.; high carbon steel: 65, 70, 65Mn, etc.
Dedicated Structural Steel Plates
1. Steel plates for pressure vessels: These are denoted by a capital R at the end of the grade, which can be indicated by either yield point or carbon content/alloy elements. For instance, Q345R, where Q345 is the yield point. Similarly, 20R, 16MnR, 15MnVR, 15MnVNR, 8MnMoNbR, MnNiMoNbR, 15CrMoR, and others signify carbon content or alloy elements.
2. Steel plates for welding gas cylinders: These are indicated by a capital HP at the end of the grade. The grade can be represented by the yield point such as Q295HP, Q345HP, or by alloy elements like 16MnREHP.
3. Boiler steel plates: Denoted by a lowercase g at the end of the grade. The grade can be indicated by the yield point, like Q390g, or by carbon content or alloy elements, such as 20g, 22Mng, 15CrMog, 16Mng, 19Mng, 13MnNiCrMoNbg, 12Cr1MoVg, etc.
4. Bridge steel plates: Indicated by a lowercase q at the end of the grade, such as Q420q, 16Mnq, 14MnNbq, etc.
5. Steel plates for automobile beams: These are denoted by a capital L at the end of the grade, such as 09MnREL, 06TiL, 08TiL, 10TiL, 09SiVL, 16MnL, 16MnREL, etc.
Color-Coated Steel Plates
Color-coated steel plates and strips are products that use metallic strip materials as a base and apply various types of organic coatings to their surfaces. These are used in areas like construction, household appliances, steel furniture, transportation tools, etc.
The classifications and codes for steel plates and strips are as per the following table:
Classification Method | Category | Code name |
By Usage | Exterior Construction | JW |
For Building Interiors | JN | |
For Household Appliances | JD | |
By Surface Condition | Coated Plate | TC |
Printed Sheets | YH | |
Embossed Sheets | YaH | |
By Type of Coating | Exterior Polyester | WZ |
Interior Use Polyester | NZ | |
Silicon Modified Polyester | GZ | |
Exterior Use Acrylic | WB | |
Interior Use Acrylic | NB | |
Plastic Sol | SJ | |
Organic Sol | YJ | |
By Substrate Category | Cold-rolled low carbon steel strip | DL |
Small Spangle Flat Steel Strip | XP | |
Large Spangle Flat Steel Strip | DP | |
Zinc-Iron Alloy Steel Strip XT | XT | |
Electro-Galvanized Steel Strip DX | DX |
Structural Steel for Shipbuilding
Generally, shipbuilding steel refers to steel used for ship hull structures, which denotes steel materials manufactured in accordance with the construction specifications of ship classification societies. These are often ordered, produced, and sold as dedicated steel and include ship plates, structural steel, and so forth.
Currently, several major steel enterprises in our country produce it and can manufacture shipbuilding steel materials according to different national specifications as per customer needs. These include standards from countries like the United States, Norway, Japan, Germany, France, etc. The specifications are as follows:
Nationality | Specification |
China | CCs |
United States | ABS |
Germany | GL |
France | BV |
Norway | DNV |
Japan | KDK |
Britain | LR |
I. Types and Specifications
Structural steel for ship hulls is classified into strength levels based on its minimum yield point: general strength structural steel and high-strength structural steel.
General strength structural steel according to the standard specification of the China Classification Society is divided into four quality grades: A, B, D, E. High-strength structural steel according to the standard specification of the China Classification Society is divided into three strength levels, four quality grades:
A32 | A36 | A40 |
D32 | D36 | D40 |
E32 | E36 | E40 |
F32 | F36 | F40 |
II. Mechanical Properties and Chemical Composition
The mechanical properties and chemical composition of general strength structural steel for ship hulls are as follows:
Steel grade | Yield point σs (MPa) not less than | Tensile strength σ b (MPa) | Elongation σ% Not less than | Carbon C | Manganese Mn | Silicon Si | Sulfur S | Phosphorus P |
A | 235 | 400-520 | 22 | ≤ 0.21 | ≥ 2.5 | ≤ 0.5 | ≤ 0.035 | ≤ 0.035 |
B | ≤ 0.21 | ≥ 0.80 | ≤ 0.35 | |||||
D | ≤ 0.21 | ≥ 0.60 | ≤ 0.35 | |||||
E | ≤ 0.18 | ≥ 0.70 | ≤ 0.35 |
Mechanical Properties and Chemical Composition of High-Strength Hull Structural Steel
Steel grade | Yield point σs (MPa) Not less than | Tensile strength σb (MPa) | Elongation σ% Not less than | Carbon C | Manganese Mn | Silicon Si | Sulfur S | Phosphorus P |
A32 | 315 | 440-570 | 22 | ≤0.18 | ≥0.9-1.60 | ≤0.50 | ≤0.035 | ≤0.035 |
D32 | ||||||||
E32 | ||||||||
F32 | ≤0.16 | ≤0.025 | ≤0.025 | |||||
A36 | 355 | 490-630 | 21 | ≤0.18 | ≤0.035 | ≤0.035 | ||
D36 | ||||||||
E36 | ||||||||
F36 | ≤0.16 | ≤0.025 | ≤0.025 | |||||
A40 | 390 | 510-660 | 20 | ≤0.18 | ≤0.035 | ≤0.035 | ||
D40 | ||||||||
E40 | ||||||||
F40 | ≤0.16 | ≤0.025 | ≤0.025 |
III. Points to Note for Delivery and Acceptance of Shipbuilding Steel:
Review of quality certification:
Upon delivery, the steel mill will certainly deliver and provide original quality certificates according to the user’s requirements and the contract’s agreed-upon specifications. The certificate must include the following content:
(1) Required specifications;
(2) Quality record number and certification number;
(3) Batch number and technical grade;
(4) Chemical composition and mechanical properties;
(5) Certificate of approval from the ship classification society and the signature of the ship surveyor.
Physical examination:
Upon delivery of shipbuilding steel, physical objects should carry the manufacturer’s mark, among other things. Specifically, they should include:
(1) Approval mark from the ship classification society;
(2) Markings drawn with paint or attached, including technical parameters such as: batch number, standard grade, dimensions, etc;
(3) The appearance should be clean and smooth, without defects.
(1) Due to the high rigidity of the structural steel formed from high-strength plates, which has large moments of inertia and high modulus of bending resistance, especially since the application requirements necessitate pre-punching before cold bending processing, differences in material surface flatness and edge dimensions can occur.
Hence, it is necessary to add more lateral positioning devices in the design of cold bending holes for such high-strength structural steel plates.
Designing appropriate hole shapes, reasonably arranging the roller gap, and ensuring that the material entering each hole shape is not deviated can mitigate the impact of differences in material surface flatness and edge dimensions on the subsequent cold bending shape.
Another significant feature is the severe springback phenomenon of high-strength structural steel plates. Springback can lead to arc edges, requiring over-bending for correction, and it’s challenging to master the over-bending angle, which needs adjustment and correction during production debugging.
(2) Multiple forming passes are required. The main processing stage in the roller cold bending process is bending deformation.
Apart from minor thinning at the local bending angle of the product, the thickness of the deformed material is assumed to remain constant during the forming process. When designing the hole shape, it’s essential to reasonably distribute the amount of deformation, especially in the first few and subsequent passes, where the deformation amount should not be too large.
Side rollers and over-bending rollers can be used to pre-bend the profiles and align the neutral line of the profile cross-section with the neutral line of the finished profile, balancing the forces exerted on the profile and avoiding longitudinal bending.
If longitudinal bending is detected during the processing, additional rollers can be added according to the situation, particularly in the later stages.
Measures like using a straightening machine for straightening, altering the frame spacing, using support rollers, and adjusting the roll gaps for each frame can minimize or eliminate longitudinal bending. It should be noted that proficient technical skills are needed to mitigate longitudinal bending by adjusting the roll gaps of each frame.
(3) The control of the roller cold bending speed and the adjustment of the forming roller pressure should be appropriate to minimize repetitive cold bending fatigue cracks.
Appropriate lubrication and cooling should be done to further reduce the occurrence of thermal stress cracks, and control the bending radius – the bending radius should not be too small, else the product surface may crack easily.
To address the post-elongation fracture phenomenon observed in high-strength plates during the cold forming and cold bending process, optimizing the section shape, such as increasing the bend radius, reducing the cold bending angle, or enlarging the section shape, under the premise of satisfying the mechanical design requirements of the material is an effective method. This is recommended in order to meet structural design requirements.